the Creative Commons Attribution 4.0 License.
the Creative Commons Attribution 4.0 License.
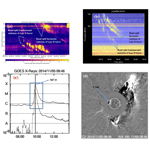
Space weather study through analysis of solar radio bursts detected by a single-station CALLISTO spectrometer
Theogene Ndacyayisenga
Ange Cynthia Umuhire
Jean Uwamahoro
Christian Monstein
This article summarises the results of an analysis of solar radio bursts (SRBs) detected by the Compound Astronomical Low-cost Low-frequency Instrument for Spectroscopy and Transportable Observatory (CALLISTO) spectrometer hosted by the University of Rwanda. The data analysed were detected during the first year (2014–2015) of the instrument operation. Using quick plots provided by the e-CALLISTO website, a total of 201 intense and well-separated solar radio bursts detected by the CALLISTO station located in Rwanda, are found consisting of 4 type II, 175 type III and 22 type IV radio bursts. It is found that all analysed type II and ∼ 37 % of type III bursts are associated with impulsive solar flares, while the minority (∼ 13 %) of type IV radio bursts are associated with solar flares. Furthermore, all type II radio bursts are associated with coronal mass ejections (CMEs), ∼ 44 % of type III bursts are associated with CMEs, and the majority (∼ 82 %) of type IV bursts were accompanied by CMEs. With aid of the atmospheric imaging assembly (AIA) images on board the Solar Dynamics Observatory (SDO), the location of open magnetic field lines of non-flare-associated type III radio bursts are shown. The same images are used to show the magnetic loops in the solar corona for type IV radio bursts observed in the absence of solar flares and/or CMEs. Findings from this study indicate that analysis of SRBs that are observed from the ground can provide a significant contribution to the early diagnosis of solar transients phenomena, such as solar flares and CMEs, which are major drivers of potential space weather hazards.
- Article
(4547 KB) - Full-text XML
- Companion paper
-
Supplement
(1847 KB) - BibTeX
- EndNote
The solar space weather events like coronal mass ejections (CMEs) and solar flares are usually accompanied by solar radio bursts (SRBs), which can be used for a low-cost real-time space weather monitoring (Lobzin et al., 2009). SRBs are classified into five types, based on their morphologies and drift rates (Wild, 1950). From the metre to decimetre range, characteristic burst signatures correspond to well-identified physical processes, such as shock waves (type II bursts; Nelson and Melrose, 1985; Cairns et al., 2003; Ganse et al., 2012), electron beams streaming along open magnetic field lines (type III bursts; Lin et al., 1981, 1986), or electron populations trapped in eruptive flux ropes and post-flare loops (type IV bursts; Nindos et al., 2008).
Type II radio bursts are the bright radio emissions often associated with CMEs and characterised by a slow-frequency drift rate (; McLean and Labrum, 1985; Nelson and Melrose, 1985). They are excited by magneto-hydrodynamics (MHD) shocks in the solar atmosphere (Nelson and Melrose, 1985; Cliver et al., 1999; Nindos et al., 2008, 2011; Vršnak and Cliver, 2008). MHD shocks are driven by both flares and CMEs in the solar atmosphere (Nindos et al., 2008). The type II radio emissions are generated at the fundamental and second harmonic of the local plasma frequency. Both the fundamental and harmonic band can be present, and sometimes each band is split into a higher and a lower frequency lane with a relative frequency splits span in the range –0.6 (Vršnak et al., 2001, 2002, 2004). Type II radio bursts that are observed in metre wavelength and associated with CMEs can serve as a proxy in estimating the CME kinematics close to the Sun (Shanmugaraju et al., 2017; Kumari et al., 2017a, b). The type III radio bursts are the intense, frequently observed and fast-drifting bursts from high to low frequencies in the dynamic spectra. These bursts usually come from active regions (Saint-Hilaire et al., 2013), and they are generated by electrons propagating along open magnetic field lines and trigger the plasma oscillations (also known as Langmuir waves) during their travel in the solar corona and interplanetary medium (IPM; e.g. Ginzburg and Zhelezniakov, 1958; Zheleznyakov, 1970; Mercier, 1975; Melrose, 1980; Pick and Ji, 1986; Saint-Hilaire et al., 2013; Sasikumar Raja and Ramesh, 2013; Reid and Ratcliffe, 2014; Mahender et al., 2020). Type III radio bursts drift from ∼ 200 to 30 MHz in less than 10 s and reach 30 kHz in about 1 h (Suzuki and Dulk, 1985; Bastian et al., 1998; Pick and Vilmer, 2008). The impulsive flares in X-ray and/or Hα wavelengths exhibit type III radio bursts at their ascending phases (Cane and Reames, 1988). The most detailed and most recent analysis of type III burst properties with their interpretations is given in the article by Reid and Ratcliffe (2014); Mahender et al. (2020) and more generally on solar radio emission (e.g. Dulk, 1985; McLean and Labrum, 1985; Bastian, 1990; Pick and Vilmer, 2008; Gary et al., 2018). On the other hand, type IV bursts are often accompanied by long-duration events observed at extreme ultraviolet radiation (EUV) or soft X-ray wavelengths and CMEs. Type IV radio bursts are broadband continuum emissions at decimetric and metric wavelengths often associated with CMEs (Pick and Ji, 1986). These bursts can have either stationary or moving sources and various emission mechanisms (Pick and Ji, 1986; Bastian et al., 1998). The main difference between moving type IV (IVm) and stationary type IV (IVs) radio bursts is that IVm shows an obvious frequency drift while IVs does not, implying that the radio source of IVm moves outward from the Sun, while IVs is stationary (Pick and Ji, 1986). Type IVs bursts since their discovery originate from plasma emission (Weiss, 1963; Benz and Tarnstrom, 1976; Salas-Matamoros and Klein, 2020). They prevail upon the presence of non-thermal electrons in the solar corona for several hours in relationship with flare and the liftoff of a CME. Additionally, other phenomena associated with flares and/or CMEs, such as small-scale ejecta (e.g. flare sprays; see Vršnak, 2001) or Moreton waves (e.g. Warmuth et al., 2001), can be sources of shocks and may accelerate particles.
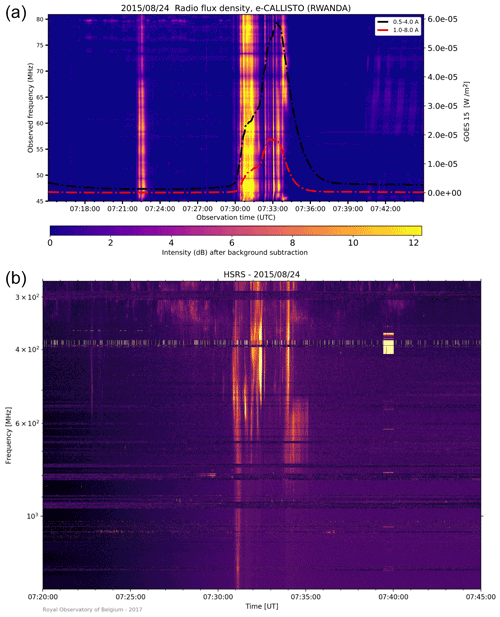
Figure 1Dynamic spectrum of 24 August 2015 type III radio bursts. (a) An isolated type III burst at 07:22 UT followed by a group of type III bursts observed at 07:30 UT by CALLISTO Rwanda. (b) A group of type III bursts observed at 07:30 UT by the ROB observatory. The one at 07:22 UT occurred during the decaying phase of a C6.7 flare (started, peaked, and ended at 07:08, 07:12 and 07:15 UT, respectively). A group of type III bursts at 07:30 UT is triggered by a M5.6 flare in its rise phase (started at 07:26 UT, peaked at 07:33 UT and ended at 07:35 UT, respectively). The black and red curves show the GOES X-ray flux.
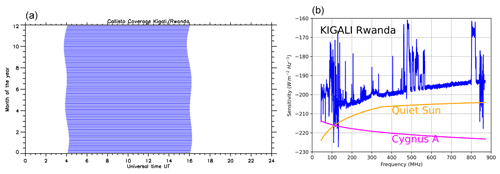
Figure 2Panel (a) shows the observation coverage by the CALLISTO station at University of Rwanda in Kigali. Panel (b) displays the spectral overview Kigali,Rwanda. Strong peaks near 100, 500 and 800 MHz are due to FM radio, digital video broadcast and mobile phone transmitters. Negative peaks with respect to the blue baseline are due to saturation of the spectrometer from strong local transmitters. The best frequencies for solar burst observations are 45–80, 150–450 and 600–800 MHz due to low interference.
Types II, III and IV radio bursts play a major role for the benefit of space weather studies because (i) their observations are used to study the underlying physics of solar eruptive events, (ii) they are often associated with space weather hazards (i.e. flares, CMEs and solar energetic particles, SEPs), and (iii) they potentially allow the study and tracking of geo-effective disturbances from the Sun through the IP medium to the Earth (e.g. Warmuth and Mann, 2004; Cane and Erickson, 2005; Miteva et al., 2017; Carley et al., 2020). CMEs and solar flares can produce streams of highly energetic particle events which can impact the Earth's magnetosphere and ionosphere and affect the performance of radio communication systems and can even influence the tropospheric weather. Adverse space weather can specifically affect satellite communication, in particular the global navigation satellite systems (GNSSs), or directly damage satellites, while various radiations can be hazardous for astronauts and crew of the flights (e.g. Carley et al., 2020; Vourlidas et al., 2020). It has been reported in the literature (Cerruti et al., 2008; Carrano et al., 2009; Muhammad et al., 2015) that the occurrence of an intense SRB can influence the GPS signal, reducing the carrier-to-noise power spectral density ratio () of all the receivers located in the sunlit hemisphere of the Earth.
It is important to mention here that X-rays, EUV flux and SEPs from the solar flares reach the ionosphere within hours and impact the high frequency (HF) communication, while CMEs take 1 to 5 d to arrive at Earth, depending on their speeds and direction. This article tends to explain how radio bursts observed from ground play a vital role in predicting space weather hazards. The diagnosis of the solar atmosphere by a better understanding of the solar radio emissions is sophisticated by ever-increasing instruments technology. With this advancement, several ground and space telescopes have been built to observe SRBs at a global scale. Space-based observations detect interplanetary bursts at ≤14 MHz using WAVES–WIND (Bougeret et al., 1995) and WAVES–STEREO (Kaiser, 2005; Rucker et al., 2005) instruments. Globally distributed ground-based solar radio spectrographs include the Radio Solar Telescope Network (RSTN) operated by U.S. Air Force (Guidice et al., 1981), the Hiraiso Radio Spectrograph (HiRAS) in Japan (Kondo et al., 1994), ARTEMIS-IV in Greece (Caroubalos et al., 2001), IZMIRAN in Russia (Gorgutsa et al., 2001), Gauribidanur Low-frequency Solar Spectrograph (GLOSS) in India (Kishore et al., 2014). The following instruments are used in the decametre wavelength: Nançay Decametre Array (Boischot et al., 1980), the Ukrainian T-shape Radio telescope model 2, UTR-2 (Braude et al., 1978; Braude, 1992), the Ukrainian Radio Interferometer of NASU, URAN (Braude et al., 1992; Megn et al., 1997), and many others (https://www.astro.gla.ac.uk/users/eduard/cesra/?page_id=187, last access: 24 July 2021). The development of the Square Kilometre Array (SKA; Dewdney et al., 2009; Nindos et al., 2019) will open a new opportunity to understand radio wave propagation. Despite this technological advancement, gaps in data were highly recognised in developing countries, especially for the African continent (Guhathakurta et al., 2013). In order to tackle these data gaps, the International Space Weather Initiative (ISWI; http://www.iswi-secretariat.org, last access: 16 March 2021) has contributed to the observation of space weather phenomena through the deployment of ground-based instruments (Haubold et al., 2010). To this end, a Compound Astronomical Low-cost Low-frequency Instrument for Spectroscopy and Transportable Observatory (CALLISTO) radio spectrometer (Benz et al., 2005, 2009) has been deployed in different parts of the globe, which led to extended CALLISTO (e-CALLISTO). The latter is designed to operate within 10–870 MHz frequency range and probe the solar corona in heliocentric distance ranging from 1 solar radius (R⊙) to 3 R⊙ (Pohjolainen et al., 2007). Through collaboration, Rwanda has been the first country in East Africa to acquire a CALLISTO spectrometer.
The current analysis presents the trends of SRBs detected by the CALLISTO station in Rwanda and discusses their correlation with solar explosive phenomena which occurred during its first year of operation. The data used cover the period from October 2014 to September 2015 within Solar Cycle 24 (SC 24). This article is structured as follows. Section 2 describes the observation of the instrument and subsequent comparison with other spectrometers. The methods used to obtain analysed data are detailed in Sect. 3. The results and discussions are presented in Sect. 4, while concluding remarks and summary are in Sect. 5.
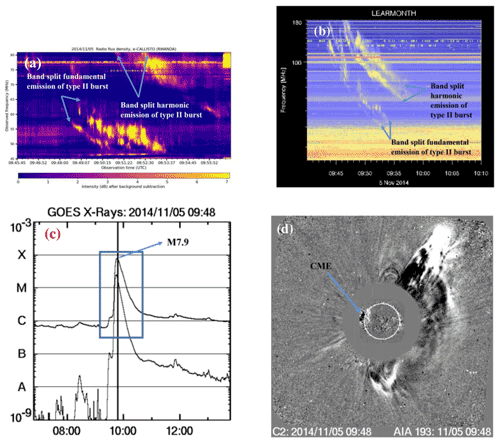
Figure 3(a) The dynamic spectrum of the 5 November 2014 type II radio burst detected by the CALLISTO station in RWANDA. (b) The 5 November 2014 type II burst observed by the Learmonth Observatory. The event has a fundamental–harmonic (F-H) structure, each with a band split due to the magnetic field. The fundamental band started at 09:48:43 UT, with 80.0 MHz, and ended at 09:53:32 UT, with 47.0 MHz, while the harmonic band started at 09:51:00 UT, with 80.9 MHz, and ended at 09:56:12 UT, with 59.0 MHz, which corresponds to the drift rates of 0.11 and 0.07 MHz s−1, respectively. (c) A M7.9 X-ray flare associated with the 5 November 2014 type II burst as detected by GOES satellite. Its start and end times are 09:26 and 09:55 UT, respectively. (d) LASCO C2 difference image shown by blue arrow. The starting time of type II burst, the flare onset and the CME are close, as shown in the images.
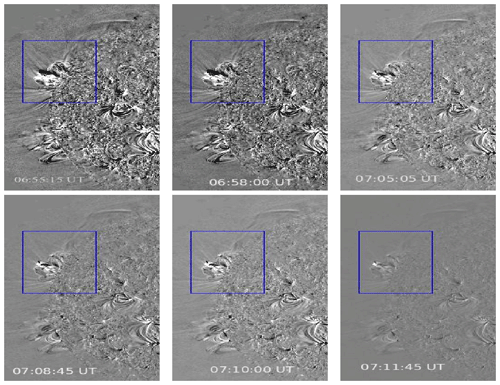
Figure 4AIA/SDO portion images of the Sun, using the 171 Å bandpass on 3 November 2014. These are running difference images corresponding to 6 type III radio bursts among 11 bursts observed on 3 November 2014 from 06:55:15 to 10:04:05 UT, respectively. This figure shows the region of the open magnetic field lines enclosed by blue rectangles which are the path of electron beams emitting type III radio bursts.
Radio burst observations using the CALLISTO spectrometer at the University of Rwanda, located in Kigali (1.9441∘ S, 30.0619∘ E), began in the year 2014, after the installation of a broadband logarithmic periodic dipole array (LPDA) and low noise amplifier (LNA; http://www.e-callisto.org/StatusReports/status20140718_V0.pdf, last access: 20 July 2021) as the primary receiving components. CALLISTO Rwanda operates within the frequency range of 45–80.9 MHz and is part of the e-CALLISTO network (Benz et al., 2005, 2009). The instrument, with a radiometric bandwidth of about 300 kHz, integration time of 1 ms and noise figure of <3 dB, allows it to detect bursts of the order of 30 SFUs (solar flux units; 1 SFU = 10−22 W m−2 Hz−1). Such a spectrometer is needed to continuously identify the nature of coherent radio emissions and to complement interferometers and array spectrometers (Bastian, 2004). The instrument is well calibrated in the time–frequency domain, such that it acquires the ability to observe radio spectra as can be seen by any other spectrometer. For instance, Fig. 1 illustrates a dynamic spectrum of type III radio bursts observed by the instrument on 24 August 2015 (top) and many others. Among the other spectrometers, the same dynamic spectrum of type III radio burst was observed by the Humain solar radio spectrometer at the Royal observatory of Belgium (ROB) that operates in frequency range from 275 to 1495 MHz and is shown as well (Fig. 1 bottom). The same event was observed by the radio telescope URAN-2 of the Poltava Gravimetric Observatory of the Geophysics Institute of the National Academy of Sciences of Ukraine (Poltava) consisting of 512 broadband dipoles. The URAN-2 operates in an 8–33 MHz frequency range (http://www.astro.gla.ac.uk/users/eduard/uran/, last access: 20 July 2021). Furthermore, this burst was also registered by Nançay Decametre Array (NDA) that operates within the 10–80 MHz frequency range.
Radio data used in this study were detected from October 2014 to September 2015 during SC 24. Using quick plots provided by the e-CALLISTO network (http://soleil.i4ds.ch/solarradio/callistoQuicklooks/, last access: 10 August 2021) and by manual inspection, we made cross-checked to identify all radio bursts observed by the CALLISTO Rwanda. As a result, a total of 201 radio bursts comprising 4 type II radio bursts, 175 type III radio bursts and 22 type IV radio bursts were identified within the 45–80.9 MHz frequency range. The time of observation varies with the seasons of the Sun. Figure 2 shows the spectral overview and the time coverage of the instrument through the year at the station.
Radio bursts are used as diagnostics of the level of solar activity (e.g. Lobzin et al., 2011; Morosan et al., 2015; Salmane et al., 2018); therefore, we carefully examined their association with the solar transients using the database from Heliophysics Event Catalogue (http://hec.helio-vo.eu/hec/hec_gui.php, last access: 12 April 2021). The latter gives white light coronagraph data from the Large Angle and Spectrometric COronagraph (LASCO C2) on board the Solar and Heliospheric Observatory (SOHO; Brueckner et al., 1995) and the Sun Earth Connection Coronal and Heliospheric Investigation (SECCHI; Howard et al., 2008) on board the Solar Terrestrial Relations Observatory (STEREO) Ahead (A) and Behind (B) spacecrafts, as well as solar flares. LASCO coronagraphs observe the Sun from 1.1–3, 2.5–6 and 4–32 R⊙ for coronagraphs 1, 2 and 3, whereas STEREO/SECCHI has two white light coronagraphs, cor 1 and cor 2, observing the Sun from 1.3–4 and 2–15 R⊙, respectively.
The association of SRBs and CMEs was confirmed upon the time coincidence (a time window of ∼ 1 h of CME from the onset of radio burst was considered). For the events where multiple CMEs occurred at the same time, we checked the source region of the moving bursts from CALLISTO images to associate them with the correct CME. In this study, a burst is considered as being flare associated if it appears between its onset and end times. For some type III and type IV bursts, it was not easy to find the driving agent, hence the Atmospheric Imaging Assembly (AIA; Lemen et al., 2012) images on board the Solar Dynamics Observatory (SDO) were used to indicate the possible launch sites. Furthermore, SDO/AIA images enable us to observe jets and their source region with a temporal and spatial resolution (Innes et al., 2011).
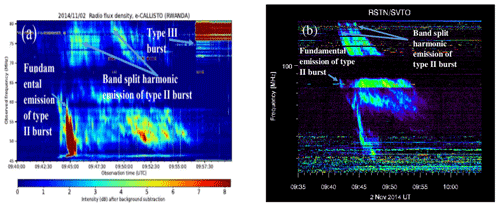
Figure 5(a) The dynamic spectrum of the 2 November 2014 type II burst followed by type III burst detected by the CALLISTO station in Rwanda. (b) The same dynamic spectrum of the 2 November 2014 burst detected from the Radio Solar Telescope Network (RSTN) at the San Vito (SVTO) station in Italy. The observed type III is of low quality, and it was only detected by CALLISTO, which proves its capacity to detect radio emissions.
4.1 Statistical analysis of observed radio bursts
The observed bursts and the associated flare properties are split into three tables according to their typical morphology. Table 1 presents four intense and well-separated type II radio bursts. Similarly, Table 2 presents type IV bursts identified, while a detailed table of type III radio bursts can be accessed at http://www.e-callisto.org/GeneralDocuments/Type_III_radio_bursts_2014_2015_kigali.pdf (last access: 25 May 2021). For each table, the analysed burst parameters, such as the burst date (yyyy/mm/dd format), the corresponding onset time and frequency range, are listed in the first four columns, respectively. The next five columns list the associated flare properties, namely the flare onset time, end times, GOES X-ray flare classes, heliographic coordinates and active regions (ARs), and the last column shows associated CME onset, respectively.
The analysis shows that all type II radio bursts are associated with solar flares, and ∼ 37 % of the type III and ∼ 13 % of type IV radio bursts detected are flare associated. Findings are compared with the results obtained in the previous similar studies using other CALLISTO stations, such as Mahender et al. (2020), who found that 426 out of 1531 (∼28 %) type III bursts were flare associated, and Ndacyayisenga et al. (2021), who inferred that 65 % of 698 type III radio studied were flare associated. The remaining non-flare-associated type III radio bursts may be due to small-scale reconnection events present in the solar corona. Furthermore, the association of these bursts with CMEs was traced out. It is observed that all analysed type II radio bursts are associated with CMEs. The obtained results agree with the recent study by Umuhire et al. (2021), who found that, among the 365 type II bursts reported on the Space Weather Prediction Center (SWPC), only 84 (23 %) were not associated with CMEs. The study found that ∼ 44 % of type III radio bursts are accompanied by CMEs. The majority (∼82 %) of type IV radio bursts are associated with CMEs, which is consistent with the results obtained by Kumari et al. (2021), who analysed a set of 446 type IV radio bursts and found that 81 % of them are temporally and spatially correlated with CMEs.
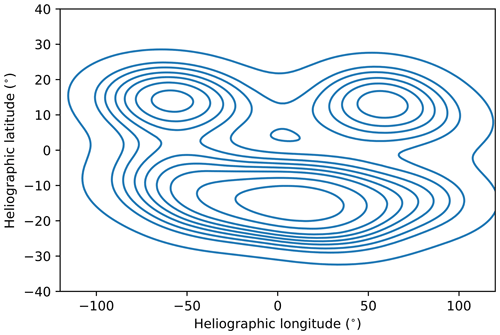
Figure 6Heliographic longitude vs. heliographic latitude of flares that have triggered the type III radio bursts.
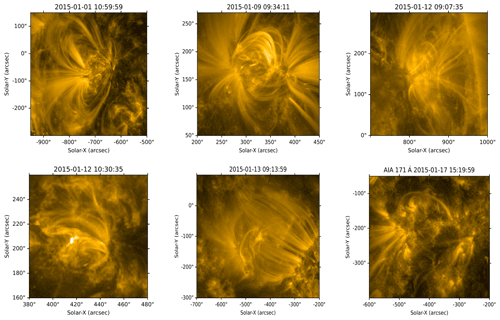
Figure 7The magnetic loops embedded in the solar corona indicating the potential site of trapped electrons responsible for type IV emissions. The figure displays a sample of six AIA/SDO images in the 171 Å band filter corresponding to the dates of type IV radio bursts in January 2015.
Among the type II bursts detected by the instrument, a case study of the 5 November 2014 event is taken. Figure 3a shows a type II radio spectrum recorded on 5 November 2014 by the CALLISTO spectrometer based in Rwanda. The type II radio burst presented in the figure shows both fundamental and harmonic band split structures. The fundamental band started at 09:48:43 UT, with 80.0 MHz, and ended at 09:53:32 UT, with 47.0 MHz, while the harmonic band started at 09:51:00 UT, with 80.9 MHz, and ended at 09:56:12 UT, with 59.0 MHz, which corresponds to the drift rates of 0.11 and 0.07 MHz s−1, respectively. The 5 November 2014 type II burst was also detected by a solar radio spectrograph located at the Learmonth Solar Observatory, Australia, among many other instruments as shown in Fig. 3b. The spectrum obtained by the two different instrument are of good quality for spectral analysis. This event was associated with a M7.9 X-ray flare (refer to Fig. 3c) that started at 09:26 UT and ended at 09:55 UT in the NOAA 12205 active region located at N15E53 on the solar disc. The 5 November 2014 burst was also associated with a partial halo CME with a linear speed of 386 km s−1, which was only detected by LASCO (C2 and C3). An illustrative CME image is shown in Fig. 3d.
A small fraction of type III bursts is found to be associated with impulsive flares. Observed radio bursts with no direct connection to impulsive flares may have originated at Hα ejecta, X-ray footpoints and X-ray or/and extreme UV (EUV) jets (Alissandrakis et al., 2015, and references therein). The date of 3 November 2014 is selected among the type III bursts reported in the table maintained at http://www.e-callisto.org/GeneralDocuments/Type_III_radio_bursts_2014_2015_kigali.pdf (last access: 25 May 2021) because a maximum number of type III radio bursts were detected on this day. Out of 12 well-separated type III radio bursts reported, only one is triggered by a solar flare. With the help of images provided by AIA/SDO, a region of open magnetic field lines as the signature of the electron stream responsible for type III radio bursts is indicated. This observation is consistent with earlier studies inferred that type III radio bursts are commonly associated with jets in extreme ultraviolet (EUV) and X-rays (e.g. Bain and Fletcher, 2009; Klassen et al., 2011; Krucker et al., 2011; Klassen et al., 2012), with typical electron beams coinciding the path as the jets. Figure 4 shows a sample of the SDO/AIA images using the 171 Å bandpass of the Sun on 3 November 2014.
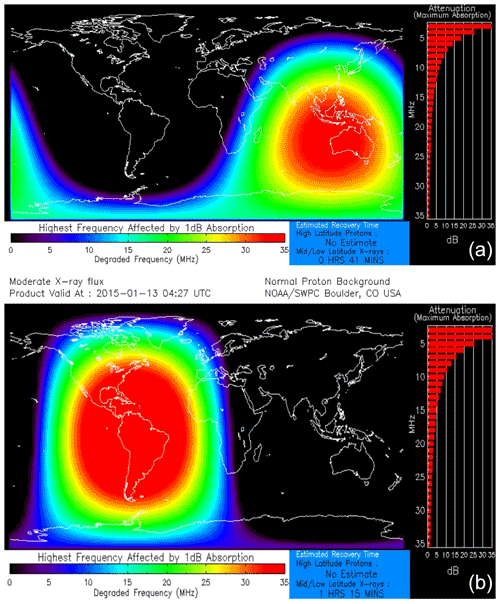
Figure 8Maps with radio blackout on 13 January 2015 (a) and on 12 March 2015 (b). Source: Space Weather Prediction Center (SWPC).
From Fig. 4, it is seen that the position of the open magnetic field lines is stationary from 06:55:15 to 10:04:05 UT, and hence, one can believe that there is a repetitive type III radio emission originating from the same source (e.g. Chifor et al., 2008). A similar trend was observed on the nine type III radio bursts observed in the absence of solar flares on 9 July 2015. On the other hand, Fig. 5a displays a dynamic spectrum of type II radio burst followed by type III radio burst of 2 November 2014, detected by CALLISTO station in Rwanda. These two types of radio bursts were associated by the C4.5 flare that started and ended at 09:20 to 10:36 UT, respectively. Figure 5b shows the same type II burst detected by San Vito (SVTO) radio spectrograph on board the RSTN located in Italy. RSTN/SVTO could not detect the followed type III burst. Even though it has a low quality (Umuhire et al., 2021), this reflects the capability of CALLISTO to detect solar radio burst in its designed frequency. The CME associated with these events was off the Sun–Earth line.
Although the small fraction of type III radio bursts, we have plotted the heliographic longitudes and latitudes of the associated solar flares as indicated in Fig. 6.
It is seen that the distribution of flares associated with type III radio bursts originate near the Sun's equator (). This result is consistent with the findings by Mahender et al. (2020), who found that the analysed 426 type III bursts associated with solar flares originating close to the equator (i.e. heliographic latitudes ).
The analysis continues with subsequent type IV radio bursts identified in the study period. Table 2 lists all type IV radio bursts observed by the instrument and their association with the solar phenomena. From this table, it can be seen that type IV bursts are highly associated with CMEs, which indicates that their presence can be used to map the location of trapped electrons and study the CME kinematics during the phases of eruption processes (Kumari et al., 2021).
We considered a case of January 2015 where a total of nine type IV radio bursts were detected, and we analysed six of them. Figure 7 presents the magnetic loops embedded in the solar corona, which is routinely the site of trapped electrons responsible for type IV emissions. The figure displays a sample of six AIA/SDO images in a 171 Å band filter corresponding to the dates of type IV radio bursts in January 2015. Recent results show that the type IV radio bursts coincide with the decaying phase of flares and/or triggered by post-eruption loops (e.g. Morosan et al., 2019; Kumari et al., 2021). Moreover, these bursts may lack both association with flares and CME eruptions (Table 2).
4.2 Space weather monitoring
In this article, we attempt to explain how SRB monitoring and analysis can be used to diagnose space weather hazards. SRBs (types II, III and IV) can be considered as being early signatures of space weather phenomena since they are often observed in association with flares and/or CMEs, which are major drivers of space weather hazards, including geomagnetic storms (e.g. Warmuth and Mann, 2004). Thereby, the detection of SRBs using ground-based instrumentation can serve as the advance warning of incoming associated solar transient events and provide insights that predict subsequent space weather hazards. For example, HF communications and the ionosphere can be disrupted by the X-ray and EUV wavelengths along with the solar energetic particles that may reach Earth within a few hours after the onset of solar flares and CMEs (e.g. Marqué et al., 2018). Type IV bursts are attributed to electrons trapped in closed field lines in the post-flare arcades and have a high degree of association with solar energetic particle events (White, 2007). Thus, they can be used as extreme solar radiation precursors. An example is extreme UV radiation on 13 January 2015 from the flare which ionised Earth's atmosphere and caused radio blackouts in different parts of the Earth. This hazard happened while our instrument detected two type IV bursts on 12 January 2015 and one on the next day. Affected areas are shown at the top of Fig. 8. Another example of radio blackout was observed on 12 March 2015 when three stations of e-CALLISTO network observed a long-lived type IV radio burst by the Krim station (Laboratory of Radio Astronomy of the Crimean Atomic Energy Station, CrAO, Ukraine) from 04:10 to 04:40 UT, followed by type III radio burst at 04:43 UT (Ukraine) and at 11:25 UT (Rwanda), respectively. The disturbances caused by X-ray enhancement are illustrated in the bottom of Fig. 8.
There are more than 150 e-CALLISTO spectrometers installed around the world that operate simultaneously. This makes the e-CALLISTO network the second to the USAF RSTN to cover the 24 h observation (e.g. Benz et al., 2009; Zucca et al., 2012; Sasikumar Raja et al., 2018). The observations from a single station can be limited by the sensitivity of the antenna. Despite technical issues, a burst can be viewed by more than one spectrometer, and this determines the uniqueness of the e-CALLISTO network. With the help of dynamic spectra provided by this network, it has been shown in a recent, related analysis (Ndacyayisenga et al., 2021) that space weather can continuously be monitored at a large scale. Therefore, a combination of information provided by each station as part of the e-CALLISTO network may contribute to revealing hidden features in space weather diagnostics.
In this study, we analysed 201 SRBs detected by the CALLISTO spectrometer installed in Kigali, Rwanda. The obtained results show that, during its first year of operation, 4 type II, 175 type III and 22 type IV radio bursts were detected in the frequency range of 45–80.9 MHz. Analysed data indicate that all detected type II radio bursts are flare associated; ∼ 37 % of observed type III bursts are triggered by impulsive flares. We found that the remaining non-flare-related type III bursts might have been triggered by small-scale features or weak energy events present in the solar corona according to the literature and with the help of AIA/SDO images in the 171 Å bandpass. All detected type II radio bursts are associated with CMEs, while only ∼ 44 % of type III are found to be accompanied by CMEs. Furthermore, we observed that the majority (∼ 82 %) of detected type IV radio bursts are accompanied by CMEs. Major findings from this study clearly indicate the importance of analysing SRBs observed from ground instrumentation in the study of space weather. More instruments for a wide coverage and regular maintenance of such instruments is recommended to monitor and predict of space weather and to supplement existing monitoring using space-borne instruments and radio interferometer arrays.
We used free data from http://e-callisto.org, last access: 10 August 2021, and we made our table of data accessible in this paper.
The supplement related to this article is available online at: https://doi.org/10.5194/angeo-39-945-2021-supplement.
TN, JU and ACU conceived of the presented idea and the design of the study. TN manually gathered the data used. CM helped in programming for data analysis. The analysis and interpretation of the results were done by TN and ACU, who later drafted the paper. This paper was critically reviewed by JU and CM for content. JU also took responsibility for overseeing the project, leading of the research activities and providing mentorship.
The contact author has declared that neither they nor their co-authors have any competing interests.
Publisher’s note: Copernicus Publications remains neutral with regard to jurisdictional claims in published maps and institutional affiliations.
This work was partially supported by the International Science Programme (ISP) through the Rwanda Astrophysics, Space and Climate Science Research Group (RASCSRG). We thank FHNW, the Institute for Data Science in Brugg/Windisch, Switzerland, for hosting the e-CALLISTO network. Our gratitude is extended to SOHO/LASCO, NOAA, GOES, SWPC and the Royal Observatory of Belgium (Brussels), RSTN, AIA/SDO, STEREO A/B, the Helioviewer (http://helioviewer.org, last access: 28 April 2021), the Poltava Gravimetrical Observatory of Geophysics (Ukraine) and the Nançay Radio Observatory (France), for making their data available online. This study also used version 2.1.5 of the SunPy open-source software package (SunPy Community et al., 2020). We also thank the team leaders Endawoke Yizengaw and Keith Groves, for the valuable discussions about part of the results that are included in this paper.
This paper was edited by Manuela Temmer and reviewed by three anonymous referees.
Alissandrakis, C. E., Nindos, A., Patsourakos, S., Kontogeorgos, A., and Tsitsipis, P.: A tiny event producing an interplanetary type III burst, Astron. Astrophys., 582, A52, https://doi.org/10.1051/0004-6361/201526265, 2015. a
Bain, H. M. and Fletcher, L.: Hard X-ray emission from a flare-related jet, Astron. Astrophys., 508, 1443–1452, https://doi.org/10.1051/0004-6361/200911876, 2009. a
Bastian, T. S.: Radio Emission from Flare Stars, Sol. Phys., 130, 265–294, https://doi.org/10.1007/BF00156794, 1990. a
Bastian, T. S.: The Frequency Agile Solar Radiotelescope, Solar and Space Weather Radiophysics. Astrophysics and Space Science Library, Vol. 314, Springer, Dordrecht, 47–69, https://doi.org/10.1007/1-4020-2814-8_3, 2004. a
Bastian, T. S., Benz, A. O., and Gary, D. E.: RADIO EMISSION FROM SOLAR FLARES, Annu. Rev. Astron. Astrophys., 36, 131–188, https://doi.org/10.1146/annurev.astro.36.1.131, 1998. a, b
Benz, A. O. and Tarnstrom, G. L.: Synchrotron or plasma process emission in narrow-band type IVdm bursts?, Astrophys. J., 204, 597–603, https://doi.org/10.1086/154208, 1976. a
Benz, A. O., Monstein, C., and Meyer, H.: Callisto A New Concept for Solar Radio Spectrometers, Sol. Phys., 226, 143–151, https://doi.org/10.1007/s11207-005-5688-9, 2005. a, b
Benz, A. O., Monstein, C., Meyer, H., Manoharan, P. K., Ramesh, R., Altyntsev, A., Lara, A., Paez, J., and Cho, K. S.: A World-Wide Net of Solar Radio Spectrometers: e-CALLISTO, Earth Moon Planet., 104, 277–285, https://doi.org/10.1007/s11038-008-9267-6, 2009. a, b, c
Boischot, A., Rosolen, C., Aubier, M. G., Daigne, G., Genova, F., Leblanc, Y., Lecacheux, A., de La Noe, J., and Moller-Pedersen, B.: A new high-grain, broadband, steerable array to study Jovian decametric emission, International Journal of Solar System Studies, Elsevier, 43, 399–407, https://doi.org/10.1016/0019-1035(80)90185-2, 1980. a
Bougeret, J. L., Kaiser, M. L., Kellogg, P. J., Manning, R., Goetz, K., Monson, S. J., Monge, N., Friel, L., Meetre, C. A., Perche, C., Sitruk, L., and Hoang, S.: Waves: The Radio and Plasma Wave Investigation on the Wind Spacecraft, Space Sci. Rev., 71, 231–263, https://doi.org/10.1007/BF00751331, 1995. a
Braude, S. I., Men, A. V., and Sodin, L. G.: The UTR-2 decametric-wave radio telescope, Antenny, 26, 3–15, 1978. a
Braude, S. Y.: Decametric radioastronomy, Astrophysics on the Threshold of the 21st Century, 81–102, 1992. a
Braude, S. Y., Megn, A. V., Rashkovsky, S. L., Sharykin, N. K., Inyutin, G. A., Shepelev, V. A., and Konovalenko, A. A.: The Ukrainian radio interferometer system URAN for studies in decametric wavelengths band (Abstract), in: Planetary Radio Emissions III, p. 183, 1992. a
Brueckner, G. E., Howard, R. A., Koomen, M. J., Korendyke, C. M., Michels, D. J., Moses, J. D., Socker, D. G., Dere, K. P., Lamy, P. L., Llebaria, A., Bout, M. V., Schwenn, R., Simnett, G. M., Bedford, D. K., and Eyles, C. J.: The Large Angle Spectroscopic Coronagraph (LASCO), Sol. Phys., 162, 357–402, https://doi.org/10.1007/BF00733434, 1995. a
Cairns, I. H., Knock, S. A., Robinson, P. A., and Kuncic, Z.: Type II Solar Radio Bursts: Theory and Space Weather Implications, Space Sci. Rev., 107, 27–34, https://doi.org/10.1023/A:1025503201687, 2003. a
Cane, H. V. and Erickson, W. C.: Studies of Space Weather Using Solar Radio Bursts, in: From Clark Lake to the Long Wavelength Array: Bill Erickson's Radio Science, edited by: Kassim, N., Perez, M., Junor, W., and Henning, P., Vol. 345, Astronomical Society of the Pacific Conference Series, p. 133, 2005. a
Cane, H. V. and Reames, D. V.: Soft X-Ray Emissions, Meter-Wavelength Radio Bursts, and Particle Acceleration in Solar Flares, Astrophys. J., 325, 895, https://doi.org/10.1086/166060, 1988. a
Carley, E. P., Baldovin, C., Benthem, P., Bisi, M. M., Fallows, R. A., Gallagher, P. T., Olberg, M., Rothkaehl, H., Vermeulen, R., Vilmer, N., and Barnes, D.: Radio observatories and instrumentation used in space weather science and operations, J. Space Weather Space Clim., 10, 7, https://doi.org/10.1051/swsc/2020007, 2020. a, b
Caroubalos, C., Maroulis, D., Patavalis, N., Bougeret, J. L., Dumas, G., Perche, C., Alissand rakis, C., Hillaris, A., Moussas, X., Preka-Papadema, P., Kontogeorgos, A., Tsitsipis, P., and Kanelakis, G.: The New Multichannel Radiospectrograph ARTEMIS-IV/HECATE, of the University of Athens, Exp. Astron., 11, 23–32, 2001. a
Carrano, C. S., Bridgwood, C. T., and Groves, K. M.: Impacts of the December 2006 solar radio bursts on the performance of GPS, Radio Sci., 44, RS0A25, https://doi.org/10.1029/2008RS004071, 2009. a
Cerruti, A. P., Kintner Jr., P. M., Gary, D. E., Mannucci, A. J., Meyer, R. F., Doherty, P., and Coster, A. J.: Effect of intense December 2006 solar radio bursts on GPS receivers, Space Weather, 6, S10D07, https://doi.org/10.1029/2007SW000375, 2008. a
Chifor, C., Isobe, H., Mason, H. E., Hannah, I. G., Young, P. R., Del Zanna, G., Krucker, S., Ichimoto, K., Katsukawa, Y., and Yokoyama, T.: Magnetic flux cancellation associated with a recurring solar jet observed with Hinode, RHESSI, and STEREO/EUVI, Astron. Astrophys., 491, 279–288, https://doi.org/10.1051/0004-6361:200810265, 2008. a
Cliver, E. W., Webb, D. F., and Howard, R. A.: On the origin of solar metric type II bursts, Sol. Phys, 187, 89–114, https://doi.org/10.1023/A:1005115119661, 1999. a
Dewdney, P. E., Hall, P. J., Schilizzi, R. T., and Lazio, T. J. L. W.: The Square Kilometre Array, Proc. IEEE, 97, 1482–1496, 2009. a
Dulk, G. A.: Radio Emission from the Sun and Stars, Annu. Rev. Astron. Astrophys., 23, 169–224, https://doi.org/10.1146/annurev.aa.23.090185.001125, 1985. a
Ganse, U., Kilian, P., Vainio, R., and Spanier, F.: Emission of Type II Radio Bursts - Single-Beam Versus Two-Beam Scenario, Sol. Phys, 280, 551–560, https://doi.org/10.1007/s11207-012-0077-7, 2012. a
Gary, D. E., Bastian, T. S., Chen, B., Fleishman, G. D., and Glesener, L.: Radio Observations of Solar Flares, in: Science with a Next Generation Very Large Array, edited by: Murphy, E., Vol. 517, Astronomical Society of the Pacific Conference Series, p. 99, 2018. a
Ginzburg, V. L. and Zhelezniakov, V. V.: On the Possible Mechanisms of Sporadic Solar Radio Emission (Radiation in an Isotropic Plasma), Sov. Astron., 2, 653, 1958. a
Gorgutsa, R. V., Gnezdilov, A. A., Markeev, A. K., and Sobolev, D. E.: An upgrade of the izmiran's solar digital radio spectrograph: First results, Astronomical and Astrophysical Transactions, 20, 547–549, https://doi.org/10.1080/10556790108213597, 2001. a
Guhathakurta, M., Davila, J. M., and Gopalswamy, N.: The International Space Weather Initiative (ISWI), Space Weather, 11, 327–329, https://doi.org/10.1002/swe.20048, 2013. a
Guidice, D. A., Cliver, E. W., Barron, W. R., and Kahler, S.: The Air Force RSTN System, in: Bulletin of the American Astronomical Society, 13, 553, 1981. a
Haubold, H. J., Gadimova, S., and Balogh, W.: Contributions of the United Nations Office for Outer Space Affairs to the International Space Weather Initiative (ISWI), arXiv e-prints, arXiv:1011.5663, 2010. a
Howard, R. A., Moses, J. D., Vourlidas, A., Newmark, J. S., Socker, D. G., Plunkett, S. P., Korendyke, C. M., Cook, J. W., Hurley, A., Davila, J. M., Thompson, W. T., St Cyr, O. C., Mentzell, E., Mehalick, K., Lemen, J. R., Wuelser, J. P., Duncan, D. W., Tarbell, T. D., Wolfson, C. J., Moore, A., Harrison, R. A., Waltham, N. R., Lang, J., Davis, C. J., Eyles, C. J., Mapson-Menard, H., Simnett, G. M., Halain, J. P., Defise, J. M., Mazy, E., Rochus, P., Mercier, R., Ravet, M. F., Delmotte, F., Auchere, F., Delaboudiniere, J. P., Bothmer, V., Deutsch, W., Wang, D., Rich, N., Cooper, S., Stephens, V., Maahs, G., Baugh, R., McMullin, D., and Carter, T.: Sun Earth Connection Coronal and Heliospheric Investigation (SECCHI), Space Sci. Rev., 136, 67–115, https://doi.org/10.1007/s11214-008-9341-4, 2008. a
Innes, D. E., Cameron, R. H., and Solanki, S. K.: EUV jets, type III radio bursts and sunspot waves investigated using SDO/AIA observations, Astron. Astrophys., 531, L13, https://doi.org/10.1051/0004-6361/201117255, 2011. a
Kaiser, M. L.: The STEREO mission: an overview, Adv. Space Res., 36, 1483–1488, https://doi.org/10.1016/j.asr.2004.12.066, 2005. a
Kishore, P., Kathiravan, C., Ramesh, R., Rajalingam, M., and Barve, I. V.: Gauribidanur Low-Frequency Solar Spectrograph, Sol. Phys, 289, 3995–4005, https://doi.org/10.1007/s11207-014-0539-1, 2014. a
Klassen, A., Gómez-Herrero, R., and Heber, B.: Electron Spikes, Type III Radio Bursts and EUV Jets on 22 February 2010, Sol. Phys., 273, 413–419, https://doi.org/10.1007/s11207-011-9735-4, 2011. a
Klassen, A., Gómez-Herrero, R., Heber, B., Kartavykh, Y., Dröge, W., and Klein, K. L.: Solar origin of in-situ near-relativistic electron spikes observed with SEPT/STEREO, Astron. Astrophys., 542, A28, https://doi.org/10.1051/0004-6361/201118626, 2012. a
Kondo, T., Isobe, T., Igi, S., Watari, S.-I., and Tokumaru, M.: The New Solar Radio Observation System At Hiraiso, Communications Research Laboratory Review, 40, 85, 1994. a
Krucker, S., Kontar, E. P., Christe, S., Glesener, L., and Lin, R. P.: Electron acceleration associated with solar jets, Astrophys. J., 742, 82, https://doi.org/10.1088/0004-637x/742/2/82, 2011. a
Kumari, A., Ramesh, R., Kathiravan, C., and Gopalswamy, N.: New Evidence for a Coronal Mass Ejection-driven High Frequency Type II Burst near the Sun, Astrophys. J., 843, 10, https://doi.org/10.3847/1538-4357/aa72e7, 2017a. a
Kumari, A., Ramesh, R., Kathiravan, C., and Wang, T. J.: Addendum to: Strength of the Solar Coronal Magnetic Field - A Comparison of Independent Estimates Using Contemporaneous Radio and White-Light Observations, Sol. Phys, 292, 177, https://doi.org/10.1007/s11207-017-1203-3, 2017b. a
Kumari, A., Morosan, D. E., and Kilpua, E. K. J.: On the Occurrence of Type IV Solar Radio Bursts in Solar Cycle 24 and Their Association with Coronal Mass Ejections, Astrophys. J., 906, 79, https://doi.org/10.3847/1538-4357/abc878, 2021. a, b, c
Lemen, J. R., Title, A. M., Akin, D. J., Boerner, P. F., Chou, C., Drake, J. F., Duncan, D. W., Edwards, C. G., Friedlaender, F. M., Heyman, G. F., Hurlburt, N. E., Katz, N. L., Kushner, G. D., Levay, M., Lindgren, R. W., Mathur, D. P., McFeaters, E. L., Mitchell, S., Rehse, R. A., Schrijver, C. J., Springer, L. A., Stern, R. A., Tarbell, T. D., Wuelser, J.-P., Wolfson, C. J., Yanari, C., Bookbinder, J. A., Cheimets, P. N., Caldwell, D., Deluca, E. E., Gates, R., Golub, L., Park, S., Podgorski, W. A., Bush, R. I., Scherrer, P. H., Gummin, M. A., Smith, P., Auker, G., Jerram, P., Pool, P., Soufli, R., Windt, D. L., Beardsley, S., Clapp, M., Lang, J., and Waltham, N.: The Atmospheric Imaging Assembly (AIA) on the Solar Dynamics Observatory (SDO), Sol. Phys., 275, 17–40, https://doi.org/10.1007/s11207-011-9776-8, 2012. a
Lin, R. P., Potter, D. W., Gurnett, D. A., and Scarf, F. L.: Energetic electrons and plasma waves associated with a solar type III radio burst, Astrophys. J., 251, 364–373, https://doi.org/10.1086/159471, 1981. a
Lin, R. P., Levedahl, W. K., Lotko, W., Gurnett, D. A., and Scarf, F. L.: Evidence for Nonlinear Wave-Wave Interactions in Solar Type III Radio Bursts, Astrophys. J., 308, 954, https://doi.org/10.1086/164563, 1986. a
Lobzin, V., Cairns, I. H., and Robinson, P. A.: Solar Cycle Variations of the Occurrence of Coronal Type III Radio Bursts and a New Solar Activity Index, Astrophys. J., 736, L20, https://doi.org/10.1088/2041-8205/736/1/L20, 2011. a
Lobzin, V. V., Cairns, I. H., Robinson, P. A., Steward, G., and Patterson, G.: Automatic recognition of type III solar radio bursts: Automated Radio Burst Identification System method and first observations, Space Weather, 7, S04002, https://doi.org/10.1029/2008SW000425, 2009. a
Mahender, A., Sasikumar Raja, K., Ramesh, R., Panditi, V., Monstein, C., and Ganji, Y.: A Statistical Study of Low-Frequency Solar Radio Type III Bursts, Sol. Phys, 295, 153, https://doi.org/10.1007/s11207-020-01722-z, 2020. a, b, c, d
Marqué, C., Klein, K.-L., Monstein, C., Opgenoorth, H., Pulkkinen, A., Buchert, S., Krucker, S., Van Hoof, R., and Thulesen, P.: Solar radio emission as a disturbance of aeronautical radionavigation, J. Space Weather Spac., 8, A42, https://doi.org/10.1051/swsc/2018029, 2018. a
McLean, D. J. and Labrum, N. R.: Solar radiophysics : studies of emission from the sun at metre wavelengths, Cambridge University press, New York, ISBN-13: 978-0521254090, 1985. a, b
Megn, A. V., Braude, S. Y., Rashkovskiy, S. L., Sharykin, N. K., Shepelev, V. A., Inutin, G. A., Khristenko, A. D., Bulatsen, V. G., Brazhenko, A. I., Koshovyy, V. V., Romanchev, Y. V., Thesevich, V. P., and Galanin, V. P.: URAN System of the Decametric Interferometers (I), Russian Radio Physics and Radio Astronomy, 2, 385–402, 1997 (in Russian). a
Melrose, D. B.: Plasma astrohysics. Nonthermal processes in diffuse magnetized plasmas – Vol. 1: The emission, absorption and transfer of waves in plasmas; Vol. 2, Astrophys. Appl., 1980. a
Mercier, C.: Evidence of Large Scale Diverging Paths in the Solar Corona for Type III Bursts Exciters, Sol. Phys., 45, 169–179, https://doi.org/10.1007/BF00152229, 1975. a
Miteva, R., Samwel, S. W., and Krupar, V.: Solar energetic particles and radio burst emission, J. Space Weather Spac., 7, A37, https://doi.org/10.1051/swsc/2017035, 2017. a
Morosan, D. E., Gallagher, P. T., Zucca, P., O'Flannagain, A., Fallows, R., Reid, H., Magdalenić, J., Mann, G., Bisi, M. M., Kerdraon, A., Konovalenko, A. A., MacKinnon, A. L., Rucker, H. O., Thidé, B., Vocks, C., Alexov, A., Anderson, J., Asgekar, A., Avruch, I. M., Bentum, M. J., Bernardi, G., Bonafede, A., Breitling, F., Broderick, J. W., Brouw, W. N., Butcher, H. R., Ciardi, B., de Geus, E., Eislöffel, J., Falcke, H., Frieswijk, W., Garrett, M. A., Grießmeier, J., Gunst, A. W., Hessels, J. W. T., Hoeft, M., Karastergiou, A., Kondratiev, V. I., Kuper, G., van Leeuwen, J., McKay-Bukowski, D., McKean, J. P., Munk, H., Orru, E., Paas, H., Pizzo, R., Polatidis, A. G., Scaife, A. M. M., Sluman, J., Tasse, C., Toribio, M. C., Vermeulen, R., and Zarka, P.: LOFAR tied-array imaging and spectroscopy of solar S bursts, Astron. Astrophys., 580, A65, https://doi.org/10.1051/0004-6361/201526064, 2015. a
Morosan, D. E., Kilpua, E. K. J., Carley, E. P., and Monstein, C.: Variable emission mechanism of a Type IV radio burst, Astron. Astrophys., 623, A63, https://doi.org/10.1051/0004-6361/201834510, 2019. a
Muhammad, B., Alberti, V., Marassi, A., Cianca, E., and Messerotti, M.: Performance assessment of GPS receivers during the September 24, 2011 solar radio burst event, J. Space Weather Spac., 5, A32, https://doi.org/10.1051/swsc/2015034, 2015. a
Ndacyayisenga, T., Uwamahoro, J., Sasikumar Raja, K., and Monstein, C.: A statistical study of solar radio Type III bursts and space weather implication, Adv. Space Res., 67, 1425–1435, https://doi.org/10.1016/j.asr.2020.11.022, 2021. a, b
Nelson, G. J. and Melrose, D. B.: Type II bursts, Cambridge University Press, 333–359, http://hdl.handle.net/102.100.100/276592?index=1, 1985. a, b, c
Nindos, A., Aurass, H., Klein, K. L., and Trottet, G.: Radio Emission of Flares and Coronal Mass Ejections. Invited Review, Sol. Phys, 253, 3–41, https://doi.org/10.1007/s11207-008-9258-9, 2008. a, b, c
Nindos, A., Alissandrakis, C. E., Hillaris, A., and Preka-Papadema, P.: On the relationship of shock waves to flares and coronal mass ejections, Astron. Astrophys., 531, A31, https://doi.org/10.1051/0004-6361/201116799, 2011. a
Nindos, A., Kontar, E. P., and Oberoi, D.: Solar physics with the Square Kilometre Array, Adv. Space Res., 63, 1404–1424, https://doi.org/10.1016/j.asr.2018.10.023, 2019. a
Pick, M. and Ji, S. C.: Type III burst sources and electron beam injection, Sol. Phys., 107, 159–165, https://doi.org/10.1007/BF00155349, 1986. a, b, c, d
Pick, M. and Vilmer, N.: Sixty-five years of solar radioastronomy: flares, coronal mass ejections and Sun Earth connection, Astron. Astrophys. Rev., 16, 1–153, https://doi.org/10.1007/s00159-008-0013-x, 2008. a, b
Pohjolainen, S., van Driel-Gesztelyi, L., Culhane, J. L., Manoharan, P. K., and Elliott, H. A.: CME Propagation Characteristics from Radio Observations, Sol. Phys., 244, 167–188, https://doi.org/10.1007/s11207-007-9006-6, 2007. a
Reid, H. A. S. and Ratcliffe, H.: A review of solar type III radio bursts, Res. Astron. Astrophys., 14, 773–804, https://doi.org/10.1088/1674-4527/14/7/003, 2014. a, b
Rucker, H. O., Macher, W., Fischer, G., Oswald, T., Bougeret, J. L., Kaiser, M. L., and Goetz, K.: Analysis of spacecraft antenna systems: Implications for STEREO/WAVES, Adv. Space Res., 36, 1530–1533, https://doi.org/10.1016/j.asr.2005.07.060, 2005. a
Saint-Hilaire, P., Vilmer, N., and Kerdraon, A.: A Decade of Solar Type III Radio Bursts Observed by the Nançay Radioheliograph 1998–2008, Astrophys. J., 762, 60, https://doi.org/10.1088/0004-637X/762/1/60, 2013. a, b
Salas-Matamoros, C. and Klein, K.-L.: Polarisation and source structure of solar stationary type IV radio bursts, Astron. Astrophys., 639, A102, https://doi.org/10.1051/0004-6361/202037989, 2020. a
Salmane, H., Weber, R., Abed-Meraim, K., Klein, K.-L., and Bonnin, X.: A method for the automated detection of solar radio bursts in dynamic spectra, J. Space Weather Spac., 8, A43, https://doi.org/10.1051/swsc/2018028, 2018. a
Sasikumar Raja, K. and Ramesh, R.: Low-frequency Observations of Transient Quasi-periodic Radio Emission from the Solar Atmosphere, Astrophys. J., 775, 38, https://doi.org/10.1088/0004-637X/775/1/38, 2013. a
Sasikumar Raja, K., Subramanian, P., Ananthakrishnan, S., and Monstein, C.: CALLISTO Spectrometer at IISER-Pune, arXiv e-prints, arXiv:1801.03547, 2018. a
Shanmugaraju, A., Bendict Lawrance, M., Moon, Y. J., Lee, J.-O., and Suresh, K.: Heights of Coronal Mass Ejections and Shocks Inferred from Metric and DH Type II Radio Bursts, Sol. Phys, 292, 136, https://doi.org/10.1007/s11207-017-1155-7, 2017. a
SunPy Community, Barnes, W. T., Bobra, M. G., Christe, S. D., Freij, N., Hayes, L. A., Ireland, J., Mumford, S., Perez-Suarez, D., Ryan, D. F., Shih, A. Y., Chanda, P., Glogowski, K., Hewett, R., Hughitt, V. K., Hill, A., Hiware, K., Inglis, A., Kirk, M. S. F., Konge, S., Mason, J. P., Maloney, S. A., Murray, S. A., Panda, A., Park, J., Pereira, T. M. D., Reardon, K., Savage, S., Sipőcz, B. M., Stansby, D., Jain, Y., Taylor, G., Yadav, T., Rajul, and Dang, T. K.: The SunPy Project: Open Source Development and Status of the Version 1.0 Core Package, Astrophys. J., 890, 68, https://doi.org/10.3847/1538-4357/ab4f7a, 2020. a
Suzuki, S. and Dulk, G.: Solar Radiophysics, Cambridge, Cambridge University Press, 289–295, 1985. a
Umuhire, A., Uwamahoro, J., Sasikumar Raja, K., Kumari, A., and Monstein, C.: Trends and characteristics of high-frequency type II bursts detected by CALLISTO spectrometers, Adv. Space Res., 68, 3464–3477, https://doi.org/10.1016/j.asr.2021.06.029, 2021. a, b
Vourlidas, A., Carley, E. P., and Vilmer, N.: Radio Observations of Coronal Mass Ejections: Space Weather Aspects, Front. Astron. Space Sci., 7, 43, https://doi.org/10.3389/fspas.2020.00043, 2020. a
Vršnak, B.: Solar flares and coronal shock waves, J. Geophys. Res, 106, 25291–25300, https://doi.org/10.1029/2000JA004009, 2001. a
Vršnak, B. and Cliver, E. W.: Origin of Coronal Shock Waves. Invited Review, Sol. Phys, 253, 215–235, https://doi.org/10.1007/s11207-008-9241-5, 2008. a
Vršnak, B., Aurass, H., Magdalenić, J., and Gopalswamy, N.: Band-splitting of coronal and interplanetary type II bursts. I. Basic properties, Astron. Astrophys., 377, 321–329, https://doi.org/10.1051/0004-6361:20011067, 2001. a
Vršnak, B., Magdalenić, J., Aurass, H., and Mann, G.: Band-splitting of coronal and interplanetary type II bursts, II. Coronal magnetic field and Alfvén velocity, Astron. Astrophys., 396, 673–682, https://doi.org/10.1051/0004-6361:20021413, 2002. a
Vršnak, B., Magdalenić, J., and Zlobec, P.: Band-splitting of coronal and interplanetary type II bursts, III. Physical conditions in the upper corona and interplanetary space, Astron. Astrophys., 413, 753–763, https://doi.org/10.1051/0004-6361:20034060, 2004. a
Warmuth, A. and Mann, G.: The Application of Radio Diagnostics to the Study of the Solar Drivers of Space Weather, Space Weather, 656, 49–68, https://doi.org/10.1007/978-3-540-31534-6_3, 2004. a, b
Warmuth, A., Vršnak, B., Aurass, H., and Hanslmeier, A.: Evolution of Two EIT/Hα Moreton Waves, Astrophys. J., 560, L105–L109, https://doi.org/10.1086/324055, 2001. a
Weiss, A. A.: The Type IV Solar Radio Burst at Metre Wavelengths, Austr. J. Phys., 16, 526, https://doi.org/10.1071/PH630526, 1963. a
White, S.: Solar Radio Bursts and Space Weather, Asian J. Phys., 16, 2007. a
Wild, J. P.: Observations of the Spectrum of High-Intensity Solar Radiation at Metre Wavelengths, III. Isolated Bursts, Austr. J. Sci. Res. A, 3, 541, 1950. a
Zheleznyakov, V. V.: Radio emission of the sun and planets, Int. Ser. Monogr. Natural Philos., 25, 150–712, 1970. a
Zucca, P., Carley, E. P., McCauley, J., Gallagher, P. T., Monstein, C., and McAteer, R. T. J.: Observations of Low Frequency Solar Radio Bursts from the Rosse Solar-Terrestrial Observatory, Sol. Phys., 280, 591–602, https://doi.org/10.1007/s11207-012-9992-x, 2012. a