the Creative Commons Attribution 4.0 License.
the Creative Commons Attribution 4.0 License.
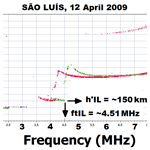
Climatology of intermediate descending layers (or 150 km echoes) over the equatorial and low-latitude regions of Brazil during the deep solar minimum of 2009
Ângela Machado dos Santos
Inez Staciarini Batista
Mangalathayil Ali Abdu
José Humberto Andrade Sobral
Jonas Rodrigues de Souza
Christiano Garnett Marques Brum
In this work, we have performed a study for the first time on the climatology of the intermediate descending layers (ILs) over Brazilian equatorial and low-latitude regions during the extreme solar minimum period of 2009. The result of this study shows that the occurrence frequency of the ILs is very high, being > 60 % over São Luís (2∘ S, 44∘ W; inclination: −3.8∘) and > 90 % in Cachoeira Paulista (22.42∘ S, 45∘ W; inclination: −33.5∘). In most cases the ILs occur during the day at altitudes varying from 130 to 180 km and they may descend to lower altitudes (∼100 km) in a time interval of a few minutes to hours. The main driving force for the ILs at the low-latitude region, may be considered to be the diurnal tide (24 h) followed in smaller dominance by the semidiurnal (12 h), terdiurnal (8 h) and quarter-diurnal (6 h) components. In the magnetic equatorial sector, similar behavior was seen, with the exception of the semidiurnal tide, which in general does not appear to have influenced the IL's dynamics (except in summer). Additionally, the IL mean descent velocity over São Luís and Cachoeira Paulista shows a day-to-day variability that may be associated with a wave-like perturbation with a periodicity of some days. Some peculiarities in the IL dynamics were noted, such as the presence of the ILs during the night hours. Ascending and descending ILs appeared to have been formed from some connection with the ionospheric F layer. Quite often, these characteristics are observed in the presence of strong signatures of the gravity wave propagation as suggested by the F layer traces in the ionogram. The descending intermediate layer over Brazil appears to have been formed through a process of F1 layer base detachment. An interesting case study showed that an ascending ILs, initially detected at ∼130 km, reached the base of the F2 layer, due probably to the gravity wave propagation and/or the effect of a prompt penetration electric field.
- Article
(27471 KB) - Full-text XML
- BibTeX
- EndNote
The first observations on the existence of intermediate layers were reported in 1930s (Schafer and Goodall, 1933; Appleton, 1933; Ratcliffe and White, 1933). It was observed that this ionization layer, which is located between the E and F1 layers, occurred regularly in the height region of around 130–150 km. Since then, some studies have been carried out on the behavior of intermediate descending layers (ILs) over different longitude sectors using radar observations (Kudeki and Fawcett, 1993; Chau and Kudeki, 2006; Kudeki et al., 1998; Tsunoda and Ecklund, 2004) and ionosonde data (Rodger et al., 1981; MacDougall, 1974, 1978; Wilkinson et al., 1992; Szuszczewicz et al., 1995). Balsley (1964) showed that the existence of these layers located at ∼150 km of altitude exhibited a downward movement during the daytime and ascending movement at dusk, with the intensity of the layer varying on a timescale of 5 to 15 min. Shen et al. (1976), observed that the ILs over Arecibo lasted for several hours. They also mentioned that in the valley region, the peak electron density of the layer ranged from to 1×103 cm−3.
According to Fujitaka and Tohmatsu (1973), the S2 and S4 propagation mode of atmospheric tide could be the dominant cause of the intermediate layers at night over middle latitudes. Over the equatorial region, the ILs could possibly be caused by gravity waves (Kudeki and Fawcett, 1993). However, some characteristics observed also suggested that the phase velocity along the line-of-sight must be controlled firstly by the large-scale electrodynamics effect (driven by tides) and secondarily by the gravity waves of short period.
Some particularities of the IL such as its seasonality and cause–effect relationships have been studied over many years. Mathews and Bekeny (1979), for example, investigated the role of tidal winds in their diurnal and semidiurnal components in the formation of ILs and concluded that the tidal winds could play a key role in the generation of these layers. Using ionosonde data, MacDougall (1978) observed that the periodicity of the intermediate layers in the ionograms appeared to be related to semidiurnal oscillations. Mathews (1998) reported on the important role of the diurnal and semidiurnal tides in the generation and descent of the intermediate layers over Arecibo. Tsunoda (1994) suggested that a gravity wave wind-driven interchange instability could be a possible generation mechanism of the field-aligned plasma irregularities responsible for the echoes received from the IL.
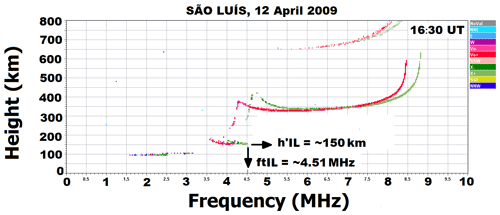
Figure 1Ionogram over São Luis on 12 April 2009. The virtual height (h′IL) and the top frequency (ftIL) of the intermediate layer are indicated in this figure. The ordinary and extraordinary traces are represented by red and green colors, respectively.
Regarding the study of the ILs using radar data, the first observations of the “150 km echoes” over the Brazilian equatorial region were made by de Paula and Hyssel (2004). The radar echoes were identified at around 09:00 LT at ∼165 km altitude, and by 12:00 LT the echoes descended to ∼145 km. A gradual upward movement of the ILs reaching 160 km of altitude was observed in the following hours. At 14:00 LT the IL disappeared, presenting in this way a local time variation resembling a “necklace” shape. A similar pattern was observed in different longitudinal sectors. From the analysis of the range–time–intensity (RTI) maps, Rodrigues et al. (2011) showed that the lowest rate of occurrence of the 150 km echoes in Brazil was during the March equinox, whereas the strongest and longest duration echoes were observed between June and September. Another important finding by Rodrigues et al. (2011) was that there was an apparent variability of the Doppler displacement with height, indicating that some of ILs over Brazil might have a different formation mechanisms from those operative in other longitudinal sectors. The ILs observed with the 30 MHz radar at São Luís presented a thickness of 3 to 5 km and were located between 140 and 170 km of altitude.
In this paper, we present for the first time the climatology of the intermediate layers over the equatorial and low-latitude locations in Brazil during a period of extremely low solar activity. The important points to be discussed in this paper include the influence of atmospheric tides and gravity waves in the IL dynamics and the possible contribution of disturbance electric fields in some specific cases. Some peculiarities found in the IL characteristics over Brazil will also be discussed.
The observational data analyzed in this work were obtained from the digisondes operated at São Luís (SL; 2∘ S, 44∘ W; I: −5.7∘) and Cachoeira Paulista(CP; 22.42∘ S, 45∘ W; I: −34.4∘) during the period of extreme solar minimum activity of 2009. The digisonde is an ionospheric radar composed by a transreceiver system that emits pulses of electromagnetic energy at frequencies ranging from 1 to 30 MHz. The electromagnetic signal is transmitted vertically to the ionosphere, with peak power of the order of 10 kW in the case of the digisonde DGS256 (over Cachoeira Paulista) and a peak power of the order of 300 W for digisonde DPS-4 (over São Luís). The echoes of the signals reflected by the ionospheric layers are registered as ionograms in the form of graphic plot of frequency versus virtual height. The digisonde data were preprocessed through the ARTIST software (Automatic Real Time Ionogram Scaler with True Height) and later manually edited by the SAO-Explorer (Standard Archive Output Format) software. For more details about digisonde, see for example Reisnish (1986) and Abdu et al. (2009b). The São Luis and Cachoeira Paulista digisonde data were acquired at a cadences of 10 and 15 min, respectively. The data over SL were analyzed from March to December and those over CP from January to December. The local times at the two sites are LT = UT−3 h. The following criteria were established in order to ubiquitously classify a certain layer as an intermediate layer:
- a.
When the critical frequency of the lower layer (extraordinary trace) exceeds the minimum frequency of the ordinary trace of F layer, the layer in question was considered as IL. Otherwise, the layer was classified as a regular E2 layer. Depending on the height of this layer, we carefully evaluated the identification of the IL case by case.
- b.
In the cases in which the characteristics described in item (a) were not clear, a sequence of ionograms was used to classify the type of the layer.
- c.
When a new layer appeared to be formed from a detachment of F1 layer, the IL was only considered when such detachment was total.
- d.
For a descending layer to be classified as IL it should first occur at or above 130 km.
- e.
When the studied layer was initially detected below 130 km, but in the sequence of the ionograms an ascending movement reaching h≥130 km was observed, the layer was classified as an IL.
- f.
The IL height and frequency parameters were extracted from the extraordinary trace. Besides that, they were processed during their downward movement until they merged into sporadic-E (Es) layers.
It is important to note that, according to the criteria mentioned above, the layers considered here as “intermediate” may have evolved into a normal c-type sporadic-E layer, for example. Figure 1 shows an example of an intermediate layer located at ∼150 km (h′IL) with a top frequency (ftIL) of 4.51 MHz. We can see that the minimum frequency of the F layer (∼4.1 MHz) was lower than the top frequency of IL, thus satisfying the criterion described in item (a).
Figure 2 presents the month-by-month variation of the percentage of occurrence of the intermediate layers over SL and CP. This analysis was performed based on the information given in Table 1. The percentage occurrence was calculated by dividing the number of the days in which ascending or descending intermediate layers were detected (regardless of whether they were observed more than once during a day or not) by the number of days of available data. The upper panel of Fig. 2 shows the statistics over SL. It is interesting to observe the high occurrence rate (above 60 %) during 2009 over this station. In July and August, the monthly occurrence reached 100 %. Over Cachoeira Paulista, the occurrence was even more pronounced throughout the period analyzed, reaching 100 % in the months of April, June, July and December.
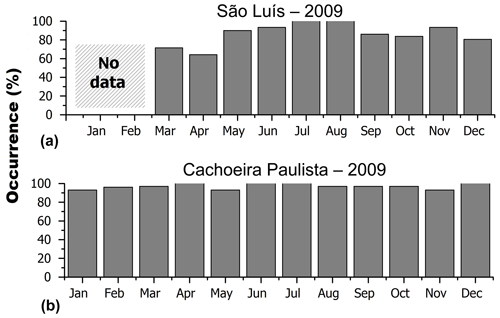
Figure 2Monthly percentage occurrence of the descending intermediate layers for solar minimum period of 2009 at São Luís (a) and Cachoeira Paulista (b). In (a) the lack of data during January and February is marked.
3.1 Seasonal and diurnal variations
Figure 3 shows mass plots of the virtual height (h′IL) and top frequency (ftIL) of the intermediate layers as a function of time. The data sets were grouped into equinoxes (March–April; September–October) and winter and summer solstices (May to August and November to February, respectively) seasons. Note that the data availability and occurrence of the ILs for these periods are summarized in Table 1. The main results of this analysis for SL and CP can be summarized as follows: (a) the IL is a phenomenon that occurs predominantly during daytime. (b) In general the higher top frequencies are observed for the intermediate layers that descend in height starting at maximum height of 130 km. (c) Over São Luís, nocturnal intermediate layers were observed between 01:00 and 08:00 UT (22:00 and 05:00 LT) in the equinoxes and summer solstice, but not in the winter during the same period. A few cases were also observed after 21:00 UT. Over CP, the nocturnal ILs were observed between ∼22:00 and 09:00 UT during the three seasons. (d) At dawn (∼06:00 LT), the lowest average top frequency of the intermediate layers over SL was ∼2.5 MHz during equinoxes and in summer, and ∼2.1 MHz in winter solstice. In CP, these values were ∼2.5 MHz in equinoxes, 2.1 MHz in summer and 1.9 MHz in winter. (e) Ascending and descending layers were observed at altitudes above 200 km during the equinoxes and winter over SL, but it is important to observe that the summer months are underrepresented due to the absence of data for January, February and some days in March. Over CP, it is possible to identify layers at altitudes slightly higher than 200 km during the three seasons. In all cases, during the interval between 05:00 and 07:00 LT (08:00–10:00 UT), the classification of the layers as ILs turned out to be difficult because this is the time during which the ionospheric E layer begins to be registered in the ionograms.
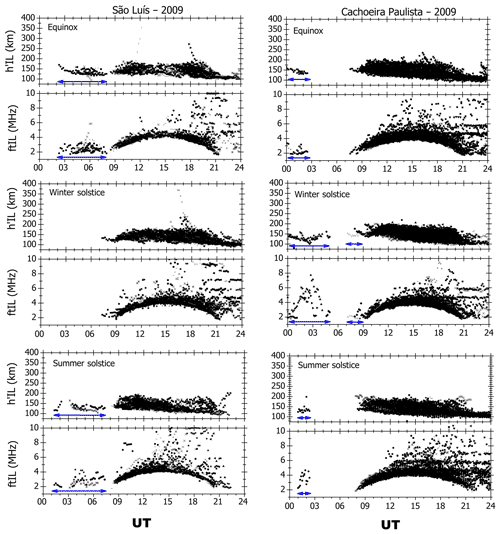
Figure 3Time dependence of intermediate layer parameters over São Luís and Cachoeira Paulista for different seasons (column of panels of left and right, respectively). The horizontal blue arrow indicates the presence of nocturnal layers between 00:00 and 09:00 UT.
Figure 4a and b show the diurnal variation of the IL parameters over SL and CP, respectively, mass-plotted for each day of the month. It is interesting to observe that in many cases, the layer descended to heights less than 130 km after ∼15:00 and 18:00 UT. In October, November and December, the layer descent started earlier, that is, soon after ∼09:00 and 13:00 UT. The ILs were not observed in March and April between 12:00 and 15:00 UT. Over CP (Fig. 4b) it is possible to note the IL tendency to reach altitudes below 130 km some hours earlier when compared with that over SL, except in November and December. In addition, in almost all the cases, the ILs were formed starting at 09:00 UT, at both SL and CP. For a better visualization, the maximum limit of the vertical axes of Fig. 4a and b was fixed at 200 km, but as can be seen in Fig. 3, on some days, the ILs were registered at altitudes well above this limit.
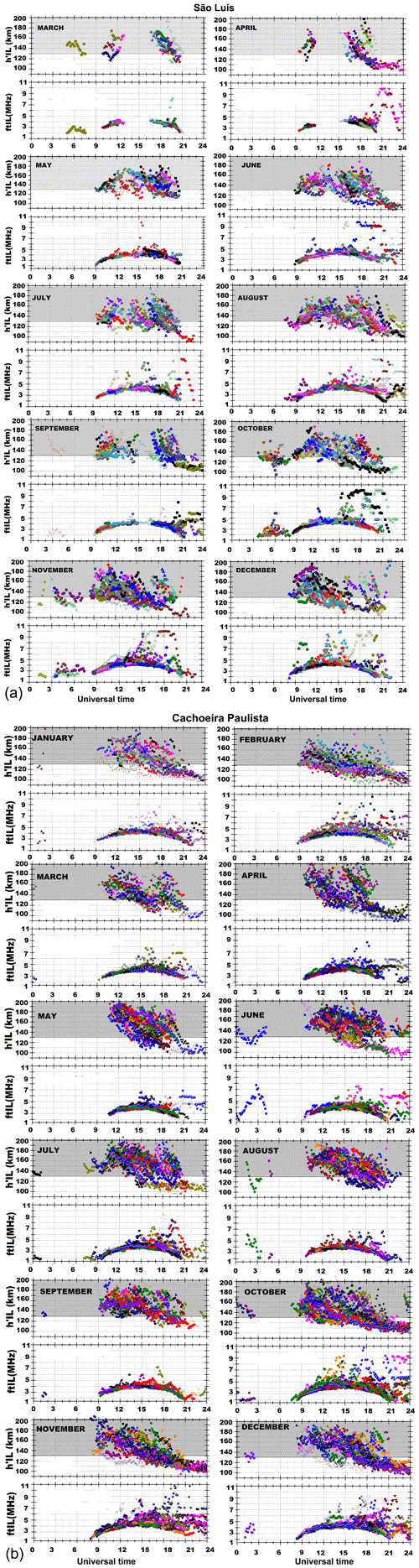
Figure 4(a) Time variation of the virtual height (h′IL) and top frequency of intermediate layers (ftIL) over São Luís from March to December 2009. Different colors are used to represent the different days of each month. (b) Same as Fig. 4a, but for Cachoeira Paulista from January to December 2009.
In general, the results presented in Fig. 4 make it clear that the height at which the ILs were detected is quite variable and the behavior of the layer top frequency is very similar to that of the E layer critical frequency with a maximum around local noon (15:00 UT). In most of the cases, the ILs present descending movements, but on some days, an ascending movement was also identified. Besides that, in our database we verified two types of ILs occurring around the same time; the ILs that may or may not descend to altitudes less than or equal to 130 km, the ILs that appear and disappear several times a day and the ILs that may be connected in some way with the F layer (that is, at h≥150 km). More details about these specific features will be given in the next sections.
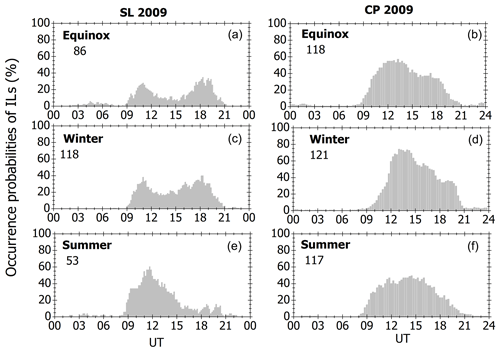
Figure 5Occurrence probability of the intermediate layer (h≥130 km) events over SL and CP during 2009 (a, c, e and b, d, f , respectively). In this plot, all the simultaneous ILs observed were considered as distinct events. The number of occurrences of IL events is indicated in each panel.
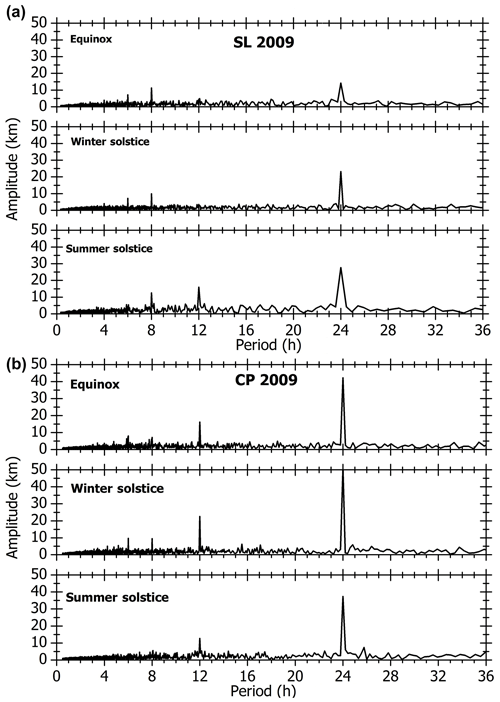
Figure 6Period amplitude of the intermediate layer heights obtained from the FFT analysis for SL (a) and CP (b) during 2009.
The analysis of the digisonde data presented here reveals, for the first time, a higher occurrence rate of the ILs during the solar minimum period with some distinct features. Previous studies have suggested that the ILs could result primarily from the wind shear processes driven by different tidal modes (Fijitaka and Tohmatsu, 1973; Mathews and Bekeny, 1979; Tong et al., 1988). Using the theoretical model for E regions (MIRE), Resende et al., 2017a, b, verified that the diurnal component of the zonal wind is very important for the formation of the Es layers (at 90–140 km) over Brazil; however, the descending movement is simulated only when the meridional wind is included in the model. Lee et al. (2003) reported that, at a Japanese location over the equatorial ionization anomaly, the semidiurnal tidal mode was dominant during spring and winter, while the quarter-diurnal mode prevailed in summer–autumn. At an Indian station, Niranjan et al. (2010) observed a high occurrence probability of the ILs during winter, moderate occurrence rate during equinox and low occurrence during summer solstice.
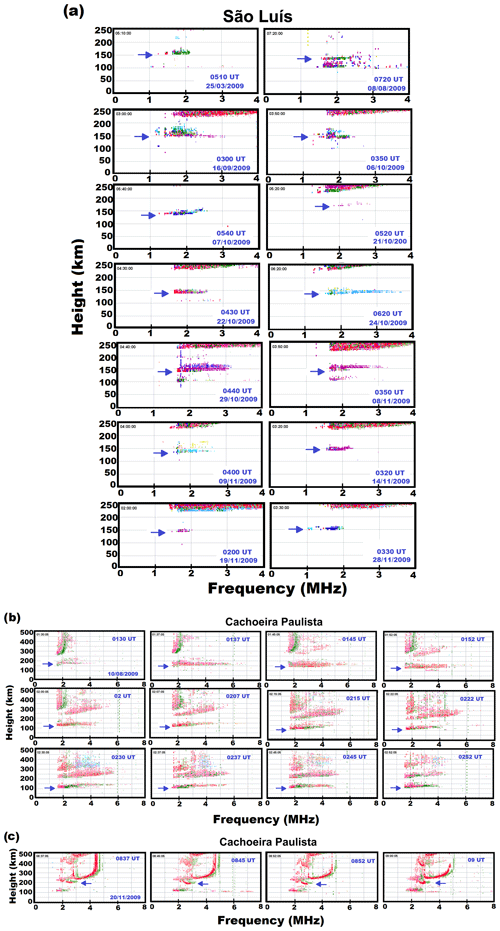
Figure 7(a) Ionograms over São Luís showing some examples of nocturnal intermediate layers on different days. (b) Same as (a), but for Cachoeira Paulista during 10 August. (c) Nocturnal intermediate layer over CP starting with a retardation in height similar to that of the h-type sporadic layer.
As presented in Fig. 3, the occurrence of ILs over the equatorial and low-latitude regions does not present any seasonal preference, but the daily occurrence probability shows an interesting perspective. Figure 5 shows the seasonally averaged local time variation of the occurrence of IL events (in percentage) for the sites in discussion. We note that during the equinoxes, the ILs occurrence above 130 km over SL shows two maxima, one at 11:00 UT and other at 18:00 UT, each one with an occurrence probability of ∼25 %–30 %. A very small occurrence probability was observed during the night (∼5 %). Further, the occurrence of the descending layers predominantly during the daytime hours in Fig. 5 appears to suggest the possibility of an important role of the E layer dynamo on the dynamics of the descending layer. A similar behavior is observed in the winter solstice with an occurrence rate of ∼35 % for both peaks. In this case, the nocturnal ILs were not observed. In the summer months, only one dominant peak was observed at 12:00 UT with an occurrence probability of 60 %. During the night, the occurrence was below 5 %. Unlike over São Luís, the maximum probability of IL occurrence over Cachoeira Paulista was characterized by two broad peaks at around 12:00 and 15:00 UT for all periods analyzed with the probability of occurrence varying between 50 % and 70 %. It is important to mention that the calculation of the occurrence probability in Fig. 5 took into consideration all the simultaneous intermediate layers' events. For this reason, there are some differences in the number of occurrences used in this analysis (indicated in each panel of Fig. 5) and those used in Fig. 2 (based in values of Table 1) because some days can have more than one IL event.
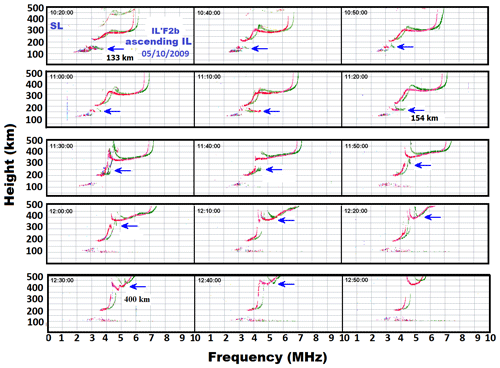
Figure 8Sequence of ionograms taken during 10:20–12:50 UT on 5 October 2009 showing the presence of ascending intermediate layers over São Luís. The blue arrow indicates more clearly the rise of IL.
It has been known from previous studies that the formation of the intermediate layers are influenced by atmospheric tides, mainly by the semidiurnal mode. In order to investigate how these waves can affect the IL dynamics over the Brazilian equatorial and low-latitude regions, we applied the fast Fourier transform (FFT) analysis on the IL height parameter and found that the semidiurnal mode could influence the ILs mainly over Cachoeira Paulista. However, the dominant influence in all the cases comes from the diurnal tide for both SL and CP. In the present analysis, the data gaps, which indicate the non-occurrence of the IL (with exceptions in January and February for SL) were replaced by the number “zero”. Figure 6 presents the result of the FFT analysis of the IL heights for São Luis (a) and for Cachoeira Paulista (b). Over São Luis, the equatorial region, the descending ILs present a well-defined diurnal periodicity in all seasons, followed by terdiurnal and quarter-diurnal modes. There is an exception in summer, when the contribution of semidiurnal mode is also evident. However, as mentioned previously, the absence of data during January and February could affect this result. Similar to the observation over SL, the role of the diurnal tide is dominant over CP (see Fig. 6b), but the influence of semidiurnal mode was evident, mainly in the equinoxes and winter. A little influence from the terdiurnal and quarter-diurnal modes can also be identified during the equinoxes and winter. As mentioned by the Niranjan et al. (2010), the ILs formation is primarily controlled by the shear forces driven by meridional and zonal winds, and their day-to-day variability (as evidenced in Fig. 4a and b) can be attributed to variations in the atmospheric tides, gravity waves, electric fields and metallic ion populations as well.
The results of Fig. 6 reveal that the influence of atmospheric tides in the formation and development of intermediate layers can have a latitudinal variation, being higher over Cachoeira Paulista than over São Luis. This behavior is in accordance with Andrioli et al. (2009a), who showed, for example, that the diurnal tidal amplitude over the low-latitude regions of Cachoeira Paulista and Santa Maria (SM; 29.7∘ S, 53.8∘ W) is higher when compared to the amplitudes over the equatorial site, São João do Cariri (7.4∘ S, 36.5∘ W) (or São Luis). Whilst the zonal component of the wind presented a semiannual variation over São João do Cariri (maximum at the equinox) and annual and semiannual variation over CP and SM, the meridional component presented a semiannual pattern over the three locations studied. Resende et al. (2017a, b) also reported that the amplitudes of the meridional and zonal winds over Cachoeira Paulista are higher than over São João do Cariri. More information about the variability of meridional and zonal winds and their diurnal and semidiurnal oscillations over Brazilian regions can be found in Batista et al. (2004), Lima et al. (2007), Buriti et al. (2008) and Andrioli et al. (2009b).
3.2 Nocturnal intermediate layers
The occurrence of nocturnal intermediate layers is one of the peculiarities found in our studies. Over São Luís, the highest number of cases was detected between October and November. Figure 7a shows some examples of the nocturnal ILs occurring between 01:20 UT (22:20 LT) and 08:30 UT (05:30 LT) over SL. The times in the ionograms do not necessarily indicate the exact moment in which the intermediate layer was formed, but they indicate the times at which they could be better visualized. It is interesting to note that in these examples, the nocturnal layers exhibited a similar shape in almost all cases, with a straight and “spreading” base appearance. The height (top frequency) of these layers varied between ∼130 and 170 km (2.2 and 3.5 MHz). Similar to the diurnal intermediate layers, the nocturnal layers also had descending and ascending characteristics.
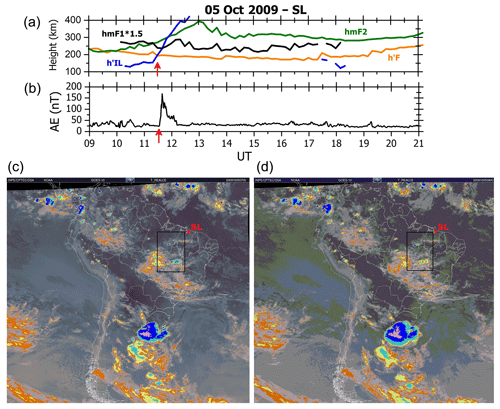
Figure 9Ascending intermediate layer over SL during 5 October. The first panel (a)–(d) shows the virtual height of the F layer (h′F, orange line), the F1 and F2 layer peak (hmF1 and hmF2, black and green lines, respectively), and the virtual height of ILs (h′IL, blue line). For a better visualization of the phenomena in discussion, the hmF1 parameter was multiplied by a factor of 1.5. The second panel shows the auroral activity index AE indicating the possible influences of prompt penetration electric field. Images from GOES satellite for 07:00 and 08:00 UT, showing the convective zones near São Luis (as indicated by the black rectangle), are shown in the last panel.
Over Cachoeira Paulista the nocturnal intermediate layers were also registered. The highest occurrence turned out to be in June and October (5 d in both months). The panels of Fig. 7b show an example of this type of layer along the night of 10 August. At around 01:30 UT an IL is observed at ∼150 km. The subsequent sequence of ionograms shows that the IL rapidly descended to ∼110 km. The range spreading characteristic was also observed in the nocturnal ILs, which in this case presented a range of ∼50 km. On the other hand, a different shape was found for the IL on 20 November, starting at 08:37 UT, as shown in the panels of Fig. 7c. We can observe at 200 km a curved format like that of the “h” type Es layer. The sequence (not presented completely here) shows that this layer descended only a few kilometers and at 10:37 UT the layer was already connected to the F1 layer.
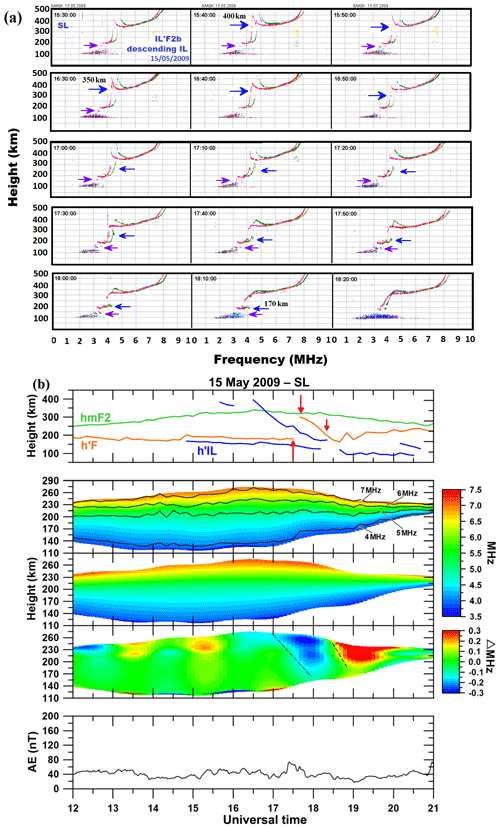
Figure 10(a) Sequence of ionograms during 15:30–18:20 UT on 15 May 2009 showing the perturbation in the F2 layer trace that reached low altitudes and evolved to a descending intermediate layer over São Luís. The blue arrow indicates the downward movement of perturbation in F layer and the purple arrow indicates the presence of another IL occurring simultaneously. (b) In the first panel the virtual height of the F layer h′F (orange line), the F2 layer peak hmF2 (green line) and the virtual height of ILs (h′IL, blue line) are shown. The red arrows indicate some modifications in the F layer in the form of the formation of the IL from a detachment of the F1 layer and the junction of the IL with the F1 layer. From the second to the fourth panels the variation of plasma frequency as a function of universal time and altitude without the high frequency (periodicities higher than 1.75 h) is shown, the reconstructed signal containing only periodicities higher than 6 h and the difference between the aforementioned profiles, respectively. The downward phase propagation in the residual ΔMHz is indicated by the dashed gray line. The last panel shows the auroral activity.
As mentioned by Lee et al. (2003), the detection of nocturnal intermediate layers may be influenced by a limitation in the digisonde, when the plasma frequency of the IL becomes lower than the lowest sounding frequency (1 MHz), or when a blanketing is caused by an underlying Es layer (l∕f type). Over Arecibo, a middle-latitude location, for example, the nocturnal IL is a very common phenomenon (Shen et al., 1976; Osterman et al., 1994). Rodger et al. (1981) also reported the presence of nocturnal ILs over South Georgia Island. The authors mentioned that the behavior of the F layer some time before the occurrence of the IL suggested that the increase in ionization observed in the region above ∼130 km and in the lower F region resulted from a downward transport from F layer probably due to the solar semidiurnal tide. According to them, the probability of occurrence of the ILs is very low when the minimum virtual height of the F2 layer base is higher than 220 km, but increases rapidly as it falls towards lower altitudes. As shown in the examples of Fig. 7a, in almost all the cases for SL, for example, we noted that the F layer was located at ∼225 km, but there are some exceptions, like that on 25 March, 8 August and 7 October. In those cases, the F region was slightly above 250 km. Over CP, the nocturnal layers had similar characteristics. On 10 August, we can see clearly that the descent of the intermediate layer occurred during an ascending of the F layer until 02:00 UT. It is also interesting to note the similarity of the IL during this day to the Es layer with high range spreading, probably formed due to particle precipitation (a-type Es layer).
3.3 Simultaneous ILs and those that are connected to the F region
Besides the occurrence of simultaneous ILs, we identify some cases in which the formation of the ILs was connected to the F region. They are (a) intermediate layers formed at the high-frequency end of the F1 layer (IL'F1t), (b) ILs formed from a detachment of the F1 layer base (IL'F1b), (c) ILs formed from a perturbation in the F2 layer base (IL'F2b) and (d) ascending ILs that reached the base of F2 layer. Among the above cited, the most common cases observed at both São Luís and Cachoeira Paulista were those in which the layers formed from a detachment of the F1 layer base.
Figure 8 shows an example of ascending intermediate layer over SL that was located initially at ∼130 km. At 11:00 UT, this layer presented a weakening followed by an intensification after 10 min. Between 10:20 and 11:20 UT, we can observe an apparent ascending movement of the IL (from 132.9 to 153.5 km) and its subsequent merging with the F1 layer at 11:30 UT. From this time on, an extra stratification at the high-frequency end of the F1 layer with an ascending structure was observed and attained the F2 layer base at 12:30 UT (400 km). It is also interesting to note the changes that occurred in the F2 layer trace during this period.
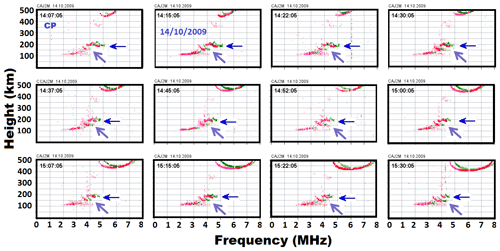
Figure 11Sequence ionograms over CP showing the presence of simultaneous intermediate layer, one being initially detected in 180 km height and the other in 150 km.
Using radar data, some authors have also shown ascending movement of the layer in the region of ∼150 km (see for example de Paula et al., 2004; Patra et al., 2007; Li et al., 2013), but hardly any discussion about them has been made until now. In order to understand the cause of this layer rise, we show in Fig. 9 some parameters of the F layer and IL during this day (5 October 2009). In Fig. 9a and b the F layer virtual height (h′F), the F1 and F2 layer peak heights (hmF1, hmF2) and the minimum virtual height of the IL trace (h′IL) are presented. Figure 9c and d present the distribution of the tropospheric convective zones as obtained from the GOES satellite above Brazil at 07:00 and 08:00 UT. We may note that at ∼11:10 UT, the IL started to rise from ∼150 km to 420 km at an interval of 1.5 h. Around the same time, the hmF2 increased but in a smoothed way. Between 12:00 and 12:40 UT, the h′IL (virtual height) was higher than hmF2 (real height). After the intermediate layer joins the F1 layer (see the ionogram at 11:30 UT in Fig. 8), the h′IL was considered as the height in which a perturbation was observed in the high-frequency end of the F1 layer until it attained the base of F2 layer. The lower two panels show images of the tropospheric convective zones (with the temperatures below −60 ∘C) around São Luis (at distances of ∼700 and 1000 km) taken some hours before the increase in the height of IL (07:00–08:00 UT), which might provide some support to the idea about the role of gravity waves in the raise of the IL. According to Vadas and Fritts (2004), the upward-propagating gravity waves excited by tropospheric convective systems could have strong impacts in the atmosphere at high altitudes due to the fact that they have long vertical wavelengths and propagate in all directions from the convection source. Besides that, some peculiarities in the ionograms during this day, in the form of the forking trace provide another evidence for the influence of the gravity waves in this event (see the last ionogram in Fig. 13). However, the increase in the AE index from 25 to 175 nT at 11:30 UT appears to have produced some effect through the action of a prompt penetration electric field (PPEF) with eastward polarity in the behavior of the IL height at this time too. It is interesting to observe that the F1 region peak height (hmF1) showed a steady increase between 11:30 and 12:15 UT while the hmF2 and h′F did not respond to this disturbance electric field. Although the hypothesis that a PPEF could have influenced the IL movement during this event, the reason for the distinct responses of the ionospheric parameters to the penetration electric field is not completed understood and would need more investigation.
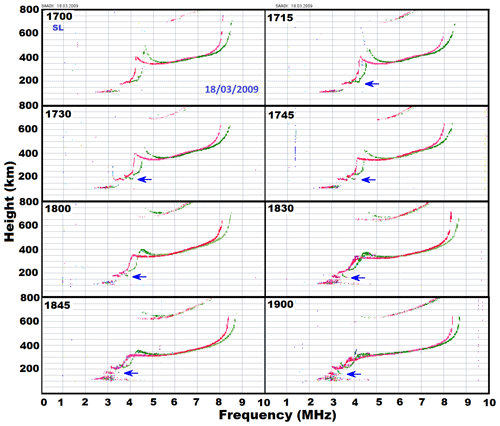
Figure 12Sequence of ionograms from SL during 18 March 2009, showing the formation of an intermediate layer from a detachment of the F1 layer base.
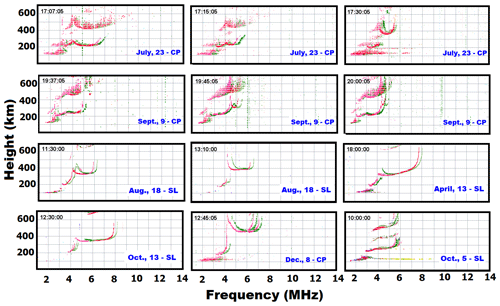
Figure 13Ionograms showing the possible manifestation of gravity waves in the intermediate layer at equatorial (SL) and low-latitude (CP) regions.
The sequence of ionograms in Fig. 8 shows that on 5 October, the IL initially detected at ∼130 km, attained first the F1 layer and continues its rise until it progressed to the F2 layer base. During this interval, considerable modifications, like a bifurcation of the F2 layer trace (see for example the ionogram from 12:10 UT), were observed. As mentioned by Abdu et al. (1982), distortions like this in the F layer trace can be a result of ionospheric disturbances induced by the atmospheric gravity waves. Besides that, our study refers to a period in which the ionosphere was considerably contracted due to the extremely low level of solar fluxes (UV, EUV and X-rays) (Heelis et al., 2009; Liu et al., 2011). Balan et al. (2012) mentioned that, under these conditions, and considering the absence of severe magnetic disturbances, the tides and waves originating in the lower atmosphere can be expected to register their effects in the thermosphere and ionosphere more easily. Using all-sky imaging, Essien et al. (2018) showed that the occurrence of small-scale (SSGWs) and medium-scale (MSGWs) gravity waves over São João do Cariri from 2000 to 2010 was higher in 2009 followed by 2008. During 2009, 289 events of SSGW and 66 events of MSGW were identified on 199 nights of observations (175 with a clear sky and 24 with clouds). These results are very interesting in that they reveal the special conditions of the coupling between the atmospheric layers during this period of deep solar minimum activity.
Figure 10 seems to present a different scenario for São Luís. As indicated by the blue arrows in Fig. 10a, an initial perturbation in the F2 layer base was observed at 15:40 UT, around 400 km. At 16:30 UT, this disturbance had already reached the F1 layer (∼350 km). The ionograms show clearly a downward movement of this stratification. At 18:10 UT, the ILs was already located at ∼170 km. It is also interesting to note the presence of a second intermediate layer (as indicated by purple arrow) that was in progress when a disturbance in the F2 layer was identified. This second layer also presented a downward movement and oscillated between periods of intensification and weakening. Figure 10b shows in the first panel the height parameters of the layers (h′F, hmF2 and h′IL). Contour plots from the upper to the bottom panels show, respectively, (1) the variation of plasma frequency as a function of universal time and altitude without the high frequency (periodicities higher than 1.75 h); (2) the reconstructed signal containing only the diurnal (24 h), semidiurnal (12 h), terdiurnal (8 h) and quarter diurnal (6 h) components; and (3) the difference between the aforementioned profiles (the residual, ΔMHz) highlighting the remaining intrinsic signal's periodicities other than 6 h > T > 1.75 h, in which T is the period. The range of frequency of these panels was limited between 4 and 7 MHz (the methodology applied to extract the variation of plasma frequency as a function of universal time and altitude based on FFT signal reconstruction is described in detail by Brum et al., 2011, and Goncharenko et al., 2013). In the last panel is shown the auroral activity index AE. Through the h′IL parameter, we can observe the occurrence of simultaneous intermediate layers on this day. During the interval in which the IL was present, a downward phase propagation in residual values of ΔMHz can be observed, as denoted by the dashed black lines mainly from 17:00 and 18:30 UT, thus characterizing the gravity waves signatures. As indicated by the red arrows in the first panel, it is also interesting to note that between 17:30 and 17:40 UT there is a discontinuity in the h′F parameter (it increases from ∼180 to 300 km). This was due to a detachment in the F1 layer base (as can be clearly seen in the ionograms of Fig. 10a) that gave origin to an IL starting from 17:40 UT. One hour later, the F1 layer became reestablished itself and at 18:30 UT the IL was connected to the F1 layer base. Similar to what happened on 5 October, in some moments the h′IL was higher than the hmF2. This occurred during the interval in which a perturbation was observed at the lower-frequency region of the F2 layer. We believe that in the absence of any magnetic disturbance, as can be verified by the very weak auroral activity, the main precursor of the IL on this day is very likely to be the gravity waves.
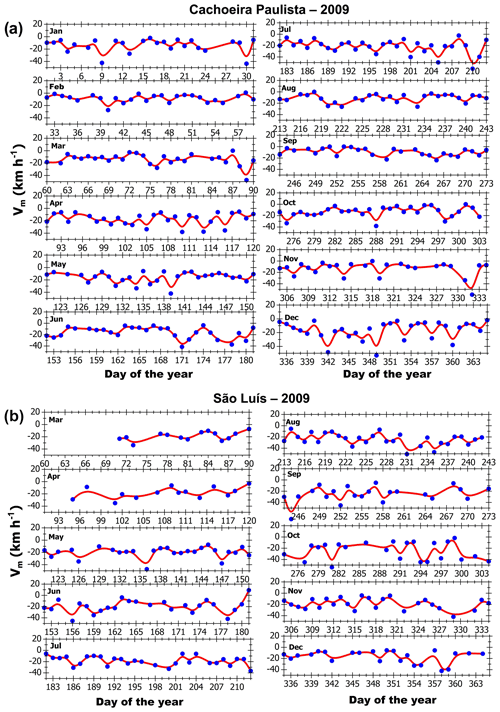
Figure 14The descending velocity of the intermediate layers for (a) Cachoeira Paulista and (b) for São Luís.
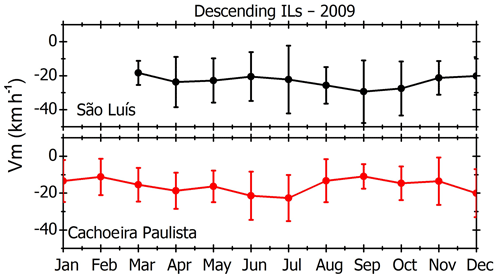
Figure 15The monthly average descending velocity of intermediate layers for São Luís and Cachoeira Paulista (black and red profiles, respectively). The vertical bars represent the standard deviation.
Figure 11 shows an example of simultaneous intermediate layers over CP. We may note that at 14:07 UT there is an IL at ∼170 km (indicated by the blue arrow) and another at 150 km (indicated by the purple arrow). In both cases, there is a very slow descent movement or nearly a stagnation of the layer at a specific height. The two layers persisted until 17:22 UT (not shown here) after which they merged at ∼130 km and continued as a single layer observed until 21:00 UT.
The occurrence of the intermediate layer arising from a detachment of the F1 layer base was the most common case found in our study. The ionograms in Fig. 12 show that at 17:15 UT on 18 March, the F1 layer base exhibited a deformation (stratification), which continued in the following ionograms. This deformation turned into a clear detachment from the F1 layer at 17:30 UT, reaching the height of ∼150 km at 19:00 UT. It is important to mention that in many other cases, a deformation in the F1 layer base was registered, but a total detachment did not occur or it occurred only later. Besides that, as mentioned previously, there were some cases in which the detachment was verified, but after some time the detached part joined the F1 layer again.
The F layer trace distortion was a frequent feature seen in our data. Figure 13 shows some examples of ionograms over the equatorial station (SL) and the low-latitude station (CP) in Brazil. Large modifications in the F1 and F2 layers were noticed, not only as bifurcations as previously mentioned, but also in the form of forking traces in both F1 and F2 layer, besides other modifications that were not very well defined. The ionograms from 13 October (SL), 8 December (CP) and 5 October (SL) show some examples of the presence of a forking trace. Note that the last example corresponds to the same day for which the case study was presented in Fig. 8. On 8 December, the digisonde registered forking traces over Cachoeria Paulista for more than 2 h. On 23 July, some interesting aspects may also be noticed. At 17:07 UT, well-defined F1 and F2 layers can be observed. Eight minutes later, the structuring of the F2 layer was considerably modified. The critical frequency decreased from ∼7.0 to ∼5.5 MHz, but the most interesting modifications were verified at 17:30 UT, when the F2 layer detached from the F1 layer and rose by ∼120 km. Later on, the sequence of ionograms (not presented here) showed that the F1 layer was transformed into a descending intermediate layer, as expected. A strong uplift of the layer in a short timescale is generally caused by a prompt penetration electric field of eastward polarity (Abdu et al., 2009a; Santos et al., 2012, 2016) but, in this case, the interplanetary magnetic field was weakly to the south ( nT) during its recovering phase. The AE and Dst indices were 150 nT (recovery phase) and −23 nT, respectively. In this scenario, we do not believe that this distortion and uplifting of the layer could be caused by a penetration electric field, because the over-shielding electric field (based on AE recovery), which is westward in this case, should make the layer go down and not rise as observed. Therefore, it is highly probable that this modification in the F2 layer and the subsequent formation of the IL was caused by a gravity wave propagation. Some oscillations were also observed over SL (not shown here), with a forking trace observed at 21:00 UT. Adding to that, Fig. 13 shows other common examples of distortions occurring in the F1 layer. Oscillations in F layer height like the one present on 18 August generally evolve into an intermediate layer detached from the F1 layer base. In another example, on 13 April, a different aspect was noted at the high-frequency end of the F1 layer. In the course of time, the characteristic that appeared to be an extra ionization evolved to a descending intermediate layer that attained heights below 130 km, for example.
3.4 Intermediate layer descent velocities
The descent velocities of the ILs for each day is presented in Fig. 14. In general, the velocities over São Luis and Cachoeira Paulista vary between −3 and −25 m s−1, except on some days in which the velocities attained values lower than −40 m s−1. Through the B-spline curve fitting, which is represented by the red curve, we may note a day-to-day variability showing that intermediate layers over both equatorial and low-latitude regions are possibly influenced by a wave-like perturbation with a periodicity of some days. The velocities obtained here are in agreement with those presented by Niranjan et al. (2010). These authors showed a case in which the IL was observed in two different intervals on the same day. In the first interval, the initial descending velocity was around 20 km h−1, which decreased to 6 km h−1 towards the end. In the second interval, the velocity was quite high, being 40 km h−1 in the first 15 min and decreasing to 8 km h−1 before it merged with the normal E layer. The daily velocities plotted in Fig. 14 were used to calculate the mean velocity for each month, which is shown in Fig. 15. We may note that the mean velocities over CP oscillated between and −23 km h−1, whilst the velocities over SL varied from −18 to −30 km h−1. It is interesting to note that the velocities over SL and CP show similar behavior from March to May. During June and July, the mean velocities over both sites were very similar, but later clear anticorrelation between the velocities can be noted.
In this work, we have presented for the first time the climatology of the 150 km echoes, or the descending intermediate layers, over the equatorial and low-latitude regions in Brazil during the extended solar minimum of 2009. The occurrence of the descending layers predominantly during daytime (Fig. 5) appears to suggest an important role played by the E layer dynamo in the dynamics of these descending layers. The present results would further suggest that this layer has a high probability of occurrence at low latitudes. The top frequency reflected by the IL presents a diurnal variation pattern similar to that of the normal E layer critical frequency, with a maximum intensity at ∼12:00 LT. The present study appears to reveal that the high occurrence rate of the IL was not related to its seasonality, mainly for CP. Further peculiarities on the layer occurrence were found, such as the presence of nocturnal layers, simultaneous ILs, ascending ILs and also their formation as a detachment from F1 layer base. It was shown from Fourier analysis that the dynamics of the ILs over the Brazilian region is dominated by the diurnal tide, followed by the semidiurnal, terdiurnal and quarter-diurnal tides. Additionally, the atmospheric gravity waves propagation and the prompt penetration of magnetospheric electric fields to equatorial latitude may both influence the dynamics of ILs.
The data used in this study may be acquired by contacting the responsible coordinators at DAE/INPE (Inez Staciarini Batista, e-mail: inez.batista@inpe.br).
AMS processed the digisonde data, performed the analysis and wrote the paper. ISB, MAA, JHAS, JRS and CGMB contributed in the interpretation of the data.
The authors declare that they have no conflict of interest.
This article is part of the special issue “7th Brazilian meeting on space geophysics and aeronomy”. It is a result of the Brazilian meeting on Space Geophysics and Aeronomy, Santa Maria/RS, Brazil, 5–9 November 2018.
Ângela M. Santos acknowledges the Fundação de Amparo à Pesquisa do Estado de São Paulo – FAPESP for the financial support under grant 2015/25357-4. Inez Staciarini Batista acknowledges CNPq support under grants 405555/2018-2 and 302920/2014-5. José Humberto Andrade Sobral has CNPq grant number 303741/2014-7. Jonas Rodrigues de Souza thanks CNPq (grant 307181/2018-9). The auroral indices were obtained from the website https://omniweb.gsfc.nasa.gov/form/omni_min.html (last access: 26 July 2019). The images from the GOES satellite were downloaded from the site: http://satelite.cptec.inpe.br/acervo/goes16.formulario.logic(last access: 25 April 2019). The Arecibo Observatory is operated by the University of Central Florida under a cooperative agreement with the National Science Foundation (AST-1744119) and in alliance with Yang Enterprises and Ana G. Méndez-Universidad Metropolitana.
This paper was edited by Igo Paulino and reviewed by two anonymous referees.
Abdu, M. A., Batista, I. S., Kantor, I. J., and Sobral, J. H. A.: Gravity Wave Induced Ionization Layers in the Night F-Region at Low Latitudes, J. Atmos. Sol.-Terr. Phys., 44, 759–767, https://doi.org/10.1016/0021-9169(82)90004-6, 1982.
Abdu, M. A., Kherani, E. A., S.Batista, I., and Sobral, J. H. A.: Equatorial evening prereversal vertical drift and spread F suppression by disturbance penetrationelectric fields, Geophys. Res. Lett., 36, L19103, https://doi.org/10.1029/2009GL039919, 2009a.
Abdu, M. A., Alam Kherani, E., Batista, I. S., de Paula, E. R., Fritts, D. C., and Sobral, J. H. A.: Gravity wave initiation of equatorial spread F/plasma bubble irregularities based on observational data from the SpreadFEx campaign, Ann. Geophys., 27, 2607–2622, https://doi.org/10.5194/angeo-27-2607-2009, 2009b.
Andrioli, V. F., Batista, P. P., Clemesha, B. R., and Schuch, N. J.: Analysis of mean winds and atmospheric tides at middle and lower latitudes in South Hemisphere, in: IAGA 2009, Sopron, Hungary, 2009a.
Andrioli, V. F., Clemesha, B. R., Batista, P. P., and Schuch, N. J.: Atmospheric tides and mean winds in the meteor region over Santa Maria (29.7∘ S; 53.8∘ W), J. Atmos. Sol.-Terr. Phys., 71, 1864–1876, 2009b.
Appleton, E. V.: Fine-Structure of the Ionosphere, Nature, 131, 872-873, 1933.
Balan, N., Chen, C. Y., Rajesh, P. K., Liu, J. Y., and Bailey, G. J.: Modeling and observations of the low latitude ionosphere-plasmasphere system at long deep solar minimum, J. Geophys. Res., 117, A08316, https://doi.org/10.1029/2012JA017846, 2012.
Balsley, B. B.: Evidence of stratified echoing region at 150 km in the 367 vicinity of magnetic equator during daylight hours, J. Geophys. Res., 69, 368–1925, https://doi.org/10.1029/JZ069i009p01925, 1964.
Batista, P. P., Clemesha, B. R., Tokumoto, A. S., and Lima, L. M.: Structure of the mean winds and tides in the meteor region over Cachoeira Paulista, Brazil (22.7∘ S; 45∘ W) and its comparison with models, J. Atmos. Sol.-Terr. Phys., 66, 623–636, https://doi.org/10.1016/j.jastp.2004.01.014, 2004.
Brum, C. G. M., Rodrigues, F. S., dos Santos, P. T., Matta, A. C., Aponte, N., Gonzalez,S. A., and Robles, E.: A modeling study of foF2 and hmF2 parameters measured by the Arecibo incoherent scatter radar and comparison with IRI model predictions for solar cycles 21, 22, and 23, J. Geophys. Res., 116, A03324, https://doi.org/10.1029/2010JA015727, 2011.
Buriti, R. A., Hocking, W. K., Batista, P. P., Medeiros, A. F., and Clemesha, B. R.: Observations of equatorial mesospheric winds over Cariri (7.4∘ S) by a meteor radar and comparison with existing models, Ann. Geophys., 26, 485–497, https://doi.org/10.5194/angeo-26-485-2008, 2008.
Chau, J. L. and Kudeki, E.: Statistics of 150-km echoes over Jicamarca based on low-power VHF observations, Ann. Geophys., 24, 1305–1310, https://doi.org/10.5194/angeo-24-1305-2006, 2006.
De Paula, E. R. and D. L. Hysell, The São Luís 30 MHz coherent scatter ionospheric radar: System description and initial results, Radio Sci., 39, RS1014, https://doi.org/10.1029/2003RS002914, 2004.
Essien, P., Paulino, I., Wrasse, C. M., Campos, J. A. V., Paulino, A. R., Medeiros, A. F., Buriti, R. A., Takahashi, H., Agyei-Yeboah, E., and Lins, A. N.: Seasonal characteristics of small- and medium-scale gravity waves in the mesosphere and lower thermosphere over the Brazilian equatorial region, Ann. Geophys., 36, 899–914, https://doi.org/10.5194/angeo-36-899-2018, 2018.
Fujitaka, K. and Tohmatsu, T.: A tidal theory of the ionosphere intermediate layer, J. Atmos. Sol.-Terr. Phys., 35, 425–438, https://doi.org/10.1016/0021-9169(73)90034-2, 1973.
Goncharenko, L. P., Hsu, V. W., Brum, C. G. M., Zhang, S.-R., and Fentzke, J. T.: Wave signatures in the midlatitude ionosphere during a sudden stratospheric warming of January 2010, J. Geophys. Res.-Space, 118, 472–487, https://doi.org/10.1029/2012JA018251, 2013.
Heelis, R. A., Coley, W. R., Burrell, A. G., Hairston, M. R., Earle, G. D., Perdue, M. D., Power, R. A., Harmon, L. L., Holt, B. J., and Lippincott, C. R.: Behavior of the O+/H+ transition height during the extreme solar minimum of 2008, Geophys. Res. Lett., 36, L00C03, https://doi.org/10.1029/2009GL038652, 2009.
Kudeki, E. and Fawcett, C. D.: High resolution observations of 150km echoes at Jicamarca, Geophys. Res. Lett., 20, 1987–1990, https://doi.org/10.1029/93GL01256, 1993.
Kudeki, E., Fawcett, C. D., Ecklund, W. L., Johnston, P. E., and Franke, S. J.: Equatorial 150-km Irregularities Observed at Pohnpei, Geophys. Res. Lett., 20, 1987–1990, https://doi.org/10.1029/1998GL900069,1998.
Lee, C.-C., Liu, J.-Y., Pan, C.-J., and Hsu, H.-H.: The intermediate layers and associated tidal motions observed by a Digisonde in the equatorial anomaly region, Ann. Geophys., 21, 1039–1045, https://doi.org/10.5194/angeo-21-1039-2003, 2003.
Li, G., Ning, B., Patra, A. K., Abdu, M. A., Chen, J., Liu, L., and Hu, L.: On the linkage of daytime 150 km echoes and abnormal intermediate layer traces over Sanya, J. Geophys. Res.-Space, 118, 7262–7267, https://doi.org/10.1002/2013JA019462, 2013.
Lima, L. M., Paulino, A. R. S., Medeiros, A. F., Buriti, R., A., Batista, P. P., Clemesha, B. R., and Takahashi, H.: First observation of the diurnal and semidiurnal ocillation in the mesospheric winds over São João doCariri-PB, Brazil, Revista Brasileira de Geofísica, 25, 35–41, 2007.
Liu, L., Chen, Y., Le, H., Kurkin, V. I., Polekh, N. M., and Lee, C.-C.: The ionosphere under extremely prolonged low solar activity, J. Geophys. Res., 116, A04320, https://doi.org/10.1029/2010JA016296, 2011.
Macdougall, J. W.: 110 km neutral zonal wind patterns, Planet. Space Sci., 22, 545–558, https://doi.org/10.1016/0032-0633(74)90089-0, 1974.
Macdougall, J. W.: Seasonal variation of semidiurnal winds in the dynamo region, Planet. Space Sci., 26, 705–714, https://doi.org/10.1016/0032-0633(78)90001-6, 1978.
Mathews, J. D.: Sporadic E: current views and recent progress, J. Atmos. Sol.-Terr. Phys., 60, 413–435, https://doi.org/10.1016/S1364-6826(97)00043-6, 1998.
Mathews, J. and Bekeny, F.: Upper atmosphere tides and the vertical motion of ionospheric sporadic layers at Arecibo, J. Geophys. Res., 84, 2743–2750, https://doi.org/10.1029/JA084iA06p02743, 1979.
Niranjan, K., Srivani, B., ad Naidu, V. V. S.: Daytime descending intermediate layers observed over a sub-tropical Indian station Waltair during low-solar activity period, Ann. Geophys., 28, 807–815, https://doi.org/10.5194/angeo-28-807-2010, 2010.
Osterman, G. B., Heelis, R. A., and Bailey, G. J.: Modeling the formation of intermediate layers at Arecibo latitudes, J. Geophys. Res., 99, 11357–11365, https://doi.org/10.1029/94JA00519, 1994.
Patra, A. K. and Rao, N. V.: Further investigations on 150-km echoing riddle using simultaneous observations of 150-km and E region echoes from off-electrojet location Gadanki, J. Geophys. Res.,112, A09301, https://doi.org/10.1029/2006JA012204, 2007.
Ratcliffe, J. A.: Fine-Structure of the Ionosphere, Nature, 131, 872–873, https://doi.org/10.1038/131872a0, 1933.
Reinisch, B. W.: New techniques in ground-based ionospheric sounding and studies, Radio Sci., 21, 331–341, 1986.
Resende, L. C. A., Batista, I. S., Denardini, C. M., Batista, P. P., Carrasco, A. J., Andrioli, V. F., and Moro, J.: Simulations of blanketing sporadic E-layer over the Brazilian sector driven by tidal winds, J. Atmos. Sol.-Terr. Phys., 154, 104–114, https://doi.org/10.1016/j.jastp.2016.12.012, 2017a.
Resende, L. C. A., Batista, I. S., Denardini, C. M., Batista, P. P., Carrasco, A. J., Andrioli, V. F., and Moro, J.: The influence of tidal winds in the formation of blanketing sporadic e-layer over equatorial Brazilian region, J. Atmos. Sol.-Terr. Phys., 171, 64–71, https://doi.org/10.1016/j.jastp.2017.06.009, 2017b.
Rodger, A. S., Fitzgerald, P. H., and Broom, S. M.: The nocturnal intermediate layer over South Georgia, J. Atmos. Sol.-Terr. Phys., 43, 1043–1050, https://doi.org/10.1016/0021-9169(81)90018-0, 1981.
Rodrigues, F. S., De Paula, E. R., and Chau, J. L.: On the characteristics of 150-km echoes observed in the Brazilian longitude sector by the 30 MHz São Luís radar, Ann. Geophys., 29, 1905–1916, https://doi.org/10.5194/angeo-29-1905-2011, 2011.
Santos, A. M., Abdu, M. A., Sobral, J. H. A., Koga, D., Nogueira, P. A. B., and Candido, C. M. N.: Strong longitudinal difference in ionospheric responses over Fortaleza (Brazil) and Jicamarca (Peru) during the January 2005 magnetic storm, dominated by northward IMF, J. Geophys. Res., 117, A08333, https://doi.org/10.1029/2012JA017604, 2012.
Santos, A. M., Abdu, M. A., Souza, J. R., Sobral, J. H. A., and Batista, I. S.: Disturbance zonal and vertical plasma drifts in the Peruvian sector during solar minimum phases, J. Geophys. Res.-Space, 121, 2503–2521, https://doi.org/10.1002/2015JA022146, 2016.
Schafer, J. P. and Goodall, W. M.: Characteristics of the Ionosphere, Nature, 131, p. 804, https://doi.org/10.1038/131804a0, 1933.
Shen, J. S., Swartz, W. S., and Farley, D. T.: Ionization layers in the nighttime E-region valley above Arecibo, J. Geophys. Res., 81, 5517–5526, https://doi.org/10.1029/JA081i031p05517, 1976.
Szuszczewicz, E. P., Roble, R. G., Wilkinson, P. J., and Hanbaba, R.: Coupling mechanisms in the lower ionospheric-thermospheric system and manifestations in the formation and dynamics of intermediate descending layers, J. Atmos. sol.-Terr. Phys., 57, 1483–1496, https://doi.org/10.1016/0021-9169(94)00145-E, 1995.
Tong, Y., Mathews, J. D., and Ying, W.-P.: An upper E region quarterdiurnal tide at Arecibo?, J. Geophys. Res., 93, 10047–10051, https://doi.org/10.1029/JA093iA09p10047, 1988.
Tsunoda, R. T.: Enhanced velocities and a shear in daytime Esq over Kwajalein and their relationship to 150km echoes over the dip equator, Geophys. Res. Let., 21, 2741–2744, https://doi.org/10.1029/94GL02507, 1994.
Tsunoda, R. T. and Ecklund, W. L.: On a summer maximum in the occurrence frequency of 150-km (F1) radar echoes over Pohnpei, Geophys. Res. Lett., 31, L06810, https://doi.org/10.1029/2003GL018704, 2004.
Vadas, S. L. and Fritts, D. C.: Thermospheric responses to gravity waves arising from mesoscale convective complexes, J. Atmos. Sol.-Terr. Phy., 66, 781–804, https://doi.org/10.1016/j.jastp.2004.01.025, 2004.
Wilkinson, P. J., Szuszczewicz, E. P., and Roble, R. G.: Measurements and modeling of intermediate, descending, and sporadic layers in the lower ionosphere: Results and implications for global-scale ionospheric-thermospheric studies, Geophys. Res. Lett., 19, 95–98, https://doi.org/10.1029/91GL02774, 1992.