the Creative Commons Attribution 4.0 License.
the Creative Commons Attribution 4.0 License.
Relationship between ionospheric plasma bubble occurrence and lightning strikes over the Amazon region
Jonas Sousasantos
José Humberto Andrade Sobral
Esfhan Alam Kherani
Marcelo Magalhães Fares Saba
Diovane Rodolfo de Campos
The vertical coupling between the troposphere and the ionosphere presents some remarkable features. Under intense tropospheric convection, gravity waves may be generated, and once they reach the ionosphere, these waves may seed instabilities and spread F and equatorial plasma bubble events may take place. Additionally, there is a close association between severe tropospheric convection and lightning strikes. In this work an investigation covering an equinox period (September–October) during the deep solar minimum (2009) presents the relation between lightning strike activity and spread F (equatorial plasma bubble) detected over a low-latitude Brazilian region. The results show a considerable correlation between these two phenomena. The common element in the center of this conformity seems to be the gravity waves. Once gravity waves and lightning strikes share the same source (intense tropospheric convection) and the effects of such gravity waves in the ionosphere include the seeding of instabilities according to the gravity waves magnitude, the monitoring of the lightning strike activity seems to offer some information about the subsequent development of spread F over the equatorial region.
Keywords. Ionosphere (equatorial ionosphere)
In recent decades several studies have been performed exposing the role of the gravity waves (GWs) on the onset and development of F region instabilities such as spread-F and equatorial plasma bubble (EPB) structures (e.g., Kherani et al., 2009, 2011; Makela et al., 2010; Vadas and Fritts, 2009; Abdu et al., 2009a; Fritts et al., 2008; Abdu, 2001; Singh et al., 1997; Huang and Kelley, 1996a–d; Huang et al., 1993; Hysell et al., 1990; Röttger, 1982; Kelley et al., 1981). spread-F and EPB events depend, in general, on a favorable unstable background configuration and the action of a seeding mechanism. During the prereversal enhancement of the zonal electric field, the vertical drift increases abruptly. Throughout this prereversal enhancement of the vertical drift (PRVD) the ionosphere is elevated and the critical parameters for the Rayleigh–Taylor instability (e.g., density gradient scale length and collision frequency) may suffer considerable modifications depending on the PRVD characteristics, in particular the value of the peak of the PRVD (Vpk). Under these conditions gravity waves reaching the ionosphere may act as a seed, triggering the instabilities which lead to the presence of spread-F and EPB structures. Under these conditions gravity waves reaching the ionosphere may act as a seed, triggering the instabilities which lead to the presence of spread-F and EPB structures.
It is widely accepted that gravity waves may be generated through tropospheric convection and thunderstorms (i.e., intense vertical cloud updrafts) (e.g., Dutta et al., 2009; Fritts et al., 2008; Hoke and Tsuda, 2001; Lane et al., 2001; Dewan et al., 1998; Taylor and Hapgood, 1988; Röttger, 1977). Additionally, the relation between lightning strikes and cloud updrafts is a known feature of the troposphere (e.g., Deierling and Petersen, 2008; Boccippio, 2001; Williams et al., 1989 and references therein). Based on these previous studies, one must expect a close relationship between lightning strike activity and the presence and generation of GW since both events share the same source.
In this work an investigation is presented which uses lightning strike data from the World Wide Lightning Location Network (WWLLN), ionospheric vertical drift, range of spread F calculated through Digisonde data and also nightglow (OI 6300 Å) images obtained with a CCD all-sky imager. An indirect relation between lightning strike activity and spread-F–EPB activity is revealed, and the common factor is argued to be the gravity waves. In the Sect. 2 the methodology of data selection and the location of the instruments are exposed. Section 3 describes the results and discussions about the dominant role of the PRVD in the spread-F–EPB phenomenology and the evidence of an external contribution of GWs in the seeding of instabilities as well as the apparent close correlation between the lightning strike activity and the GW activity in terms of time of instability onset and depleted structure evolution characteristics. The lightning strike activity monitoring seems to offer appreciable information about the intensity of the tropospheric convection and consequently the generation and presence of GWs and may be an adequate parameter to include in EPB forecasting schemes (Sousasantos et al., 2017).
For the purposes of this work, it is desirable to remove all the possible concurrent processes that may contribute to the spread-F–EPB formation (e.g., large values of Vpk and geomagnetic disturbed conditions). The year 2009 (deep solar minimum) was selected and equinoctial months (September and October) exclusively during quiet periods (Kp ≤4) were used. These conditions may provide a scenario where GWs are more robust candidates to spread-F (EPB) seeders as well as prevent the inclusion of effects such as prompt penetration electric fields and others verified during disturbed conditions (Abdu, 2012; Abdu et al., 1997, 2009b; Sobral et al., 2001, 1997). Equinoctial months present considerable EPB occurrence over the Brazilian region (see the Sobral et al., 2002, paper on climatology). On the one hand, larger values of Vpk are rarely registered, and then it seems unlikely that PRVD could potentially act as seeder (Sousasantos et al., 2013). On the other hand this ionospheric configuration points to GWs as preferable seeding sources.
The dataset is composed of 26 days ranging from 11 to 23 September 2009 (13 days) and from 11 to 23 October 2009 (13 days). These days were selected according to the availability of nightglow images (OI 6300 Å) during this period. These airglow images were registered by a CCD (charged coupled device) all-sky imager located at São João do Cariri (location given in Table 1). Additionally, Digisonde data from São Luís station (see Table 1 for location) were used to evaluate the vertical drift (and Vpk) conditions as well as to infer oscillatory motions of the ionosphere and presence of GW phase propagation in each of the days in the dataset.
The lightning counting data were obtained from WWLLN which consists of a large number of ground stations around the globe. These ground-based stations may register lightning strikes occurring inside a radius of several thousand kilometers. These lightning data were filtered in order to consider only lightning strikes occurring inside the imposed boundaries (±15∘ latitude and also ±15∘ longitude, centered in the city of Paragominas, whose location is given in Table 1). These boundaries were selected due to distribution of the WWLLN stations and the limitation in the coverage. However, this spatial window may provide data close to the region where Digisonde data and all-sky imager are located. Furthermore, we chose a time interval for monitoring the lightning activity between 15:00 and 00:00 UT (12:00–21:00 LT). This time window may appropriately accommodate different GW propagation velocities which may in turn reach ionospheric region and seed the irregularities.
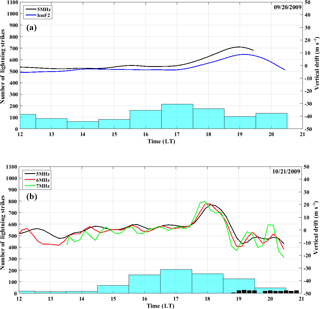
Figure 1Vertical drift (colored curves), lightning strike activity (cyan bars) and range of spread F, i.e., Qf (black bars), for 20 September 2009 (a) and 21 October 2009 (b). In (a), vertical drift was calculated through 5 MHz frequency information (altitude) and hmF2 (peak height of the F2 layer). Other frequencies were not registered on this particular day. In (b) the calculation was performed using 5, 6 and 7 MHz information.
From the Digisonde data a calculation of the vertical drift (and Vpk) was performed for the available sounding frequency data (5, 6 and 7 MHz) through d(hF)∕dt. The original data were filtered (low pass filter) with a cutoff frequency of 1 ∕ 20 min ( Hz) so that only variations with periods larger or equal to 20 min are taken into account, excluding contributions related to other processes which are not of interest for this work, since GW periods are typically in the range of 20–60 min (Fritts and Vadas, 2008). This was done for all the available frequencies used, for the entire dataset, identically. It should be mentioned that this sampling process is in complete agreement with Nyqüist's theorem, since the Digisonde data sampling period is 10 min. This procedure is similar to that of Abdu et al. (2009a).
Another parameter given by the Digisonde data was the Qf. The Qf parameter indicates the virtual range of the spread-F trace, i.e., the degree of range spreading (RSF). Abdu et al. (2012) show a clear linear relation between this parameter (RSF) and the parameter which they call “fop”, the top frequency of spread-F range. In their work, they examined both parameters and concluded that such parameters are closely related and can be used to indicate the strength of a given spread-F event.
In this study, CCD all-sky image data (OI 6300 Å nightglow emission) obtained from São João do Cariri station were used to infer the presence or absence of EPB structures. The chemical reaction involved in this emission is given by the decay of atomic oxygen and may be described as (6300 Å).
3.1 The decisive role of the prereversal enhancement of the vertical drift (PRVD)
In this section we discuss the fundamental role of the PRVD on the generation of spread-F–EPB structures. Abdu et al. (2009c) showed that over the Brazilian region, the EPB structures are majorly restricted to days where Vpk>22 m s−1. Such values of Vpk require an external seed to trigger the collisional interchange instability (CII) (general terminology for the conjunction of the gradient drift and the Rayleigh–Taylor instabilities), for which the widely accepted candidate is the gravity waves. As already mentioned, the lightning activity is somehow related to tropospheric convective activity and, thus, coincident to the GW generation. It follows that a larger number of lightning strikes indicates a region of more severe convective activity (Deierling and Petersen, 2008; Boccippio, 2001; Williams et al., 1989) and under this force of convection one can expect the generation of GWs capable of reaching the ionosphere (Dutta et al., 2009; Fritts et al., 2008; Hoke and Tsuda, 2001; Lane et al., 2001; Dewan et al., 1998; Taylor and Hapgood, 1988; Röttger, 1977).
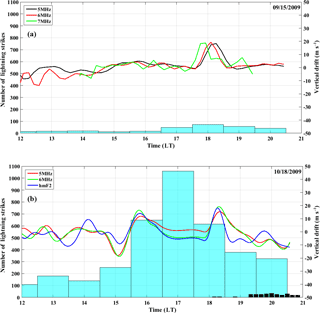
Figure 4Vertical drift (colored lines), lightning strike activity (cyan bars) and the range of spread F, Qf (black bars), for 15 September 2009 (a) and 18 October 2009 (b).
Figure 1a shows a day of the dataset in which Vpk reaches ∼15 m s−1 (20 September 2009). The black and blue curves correspond, respectively, to the vertical drifts calculated through 5 MHz frequency information (altitude) and through hmF2 (peak height of the F2 layer). This figure also presents information about the number of lightning strikes (cyan bars). Other frequencies (6 and 7 MHz) were not registered on this particular day, and for this reason a calculation through hmF2 is given as supplementary information.
The activity of lightning strikes on this day was intermediate with a slight increase in the activity a few hours (from 15:00 LT onward) before the occurrence of Vpk (∼18:50 LT). However, the absence of GWs may be noticed from the curves (5 MHz and hmF2) and no formation of spread-F–EPB structures in this day was verified either, as was also confirmed by the CCD imager data. Figure 1b shows the data for 21 October 2009. As mentioned before the filtering process applied is identical for all the data. The black, red and green curves represent, respectively, the vertical drift calculated through the frequencies of 5, 6 and 7 MHz. The cyan bars represent the lightning activity and the small black bars indicate the Qf parameter (out of scale). It should be noted that the Vpk value (∼22 m s−1) is slightly different from the previous panel (∼15 m s−1); however, the lightning strike activity is very close, indicating, by assumption, an equivalent generation, presence or absence of GWs. In this situation the fundamental role of the PRVD is clearly elucidated and the small increase in Vpk reveals the primary and critical threshold condition to the spread-F–EPB development. Again, all-sky images agree with these results, showing the presence of an EPB structure. To show the results from the CCD imager, keograms are made.
Figure 2 presents an illustration of the extraction of a keogram profile from a CCD image data. The dark color represents depleted regions while the silver color denotes regions of larger emission (density). The green dashed line represents a zonal cut in the middle of the image and the red line illustrates the profile of the emission (density) along this green dashed line. It is possible to notice that while green line crosses silver regions, the red curve shows an increase in the profile. However, when the green curve passes through dark regions, the red curve immediately reveals depletion in the profile. This depletion is the manifestation of the EPB structure as registered by the all-sky imager. Same procedure is applied for all the images and thus, a temporal profile of the structures may be constructed by normalizing each of the zonal profiles obtained. EPB structures will be manifested as large regions of depletion migrating eastward with time (Sobral et al., 1991, 1990; Abdu et al., 1985).
Figure 3 shows the results for 20 September 2009 (a) and 21 October 2009 (b). The color blue (red) indicates regions with lesser (larger) values of density as described by the color bar (right side). In Fig. 3a (20 September 2009) there are clearly no structure migrating eastward; interestingly a dark band migrating westward during the time of observation was verified, however, without any relation with spread-F–EPB events (see the absence of Qf in the Digisonde data). This unexpected event is under investigation currently.
Figure 3b (21 October 2009) reveals a large region of depletion (blue color) starting at 21:17 LT (between ∼420–650 km) and drifting eastward till ∼22:06 LT. Unfortunately, after this local time, clouds have made the observations unavailable. These panels confirm the results discussed in Fig. 1 and the prevailing role of the PRVD in the generation of spread-F–EPB structures.
3.2 Evidence for GW contribution through lightning strike activity monitoring
In the previous section a discussion about the prevailing role of the PRVD in the generation of spread/EPB structure was presented. A comparison using 2 days with similar lightning strike activity (by assumption similar GW presence or absence) shows that under the same conditions the value of Vpk is the decisive parameter for the onset and development of spread-F–EPB events. As mentioned in the introduction, it is known that lightning strike activity and severe tropospheric convection have an intrinsic relation. Also, the association of intense tropospheric convection and the generation of gravity waves was already widely discussed. In this section an investigation of some indications of GW contribution to seed instabilities leading to the subsequent development of EPB structures is presented. In addition, the approach using the lightning strike activity as a qualitative parameter for evaluation of GWs activity was found to be promising along the entire dataset.
Figure 4 shows a comparison between 15 September 2009 (a) and 18 October 2009 (b). There is a remarkable similarity in the PRVD features, especially in terms of Vpk (∼22 m s−1 for both days). However, the lightning strike activity is completely different, being almost absent during 15 September 2009 and presenting a huge number of occurrences during 18 October 2009. The vertical drift during the entire period (15:00–24:00 UT, i.e., 12:00–21:00 LT) shows an oscillatory feature on 18 October 2009 in complete agreement with the severe lightning strike activity registered, indicating the action of GW reaching the ionosphere as our hypothesis had foreseen. As discussed in the previous section, PRVD (essentially Vpk) plays a crucial role in the spread-F–EPB generation and development. However, this figure reveals a completely different scenario for extremely equivalent PRVD profiles. These discrepancies seem to be adequately explained in terms of external contributions (GW) as indicated by the huge lightning strike activity that is associated with a strong convection in the troposphere. In Fig. 4a (15 September 2009), the parameter Qf is completely absent, i.e., no spread F was registered by the Digisonde. Figure 4b (18 October 2009) shows Qf registers starting immediately after the PRVD onset (∼21:15 UT, i.e., 18:15 LT). This spread-F activity persists along the entire period of observation shown in this panel (up to 24:00 UT, i.e., 21:00 LT). It must be said that there was no 7 MHz information on 18 October 2009 (Fig. 4b); thus, hmF2 is used to obtain the vertical drift (blue curve).
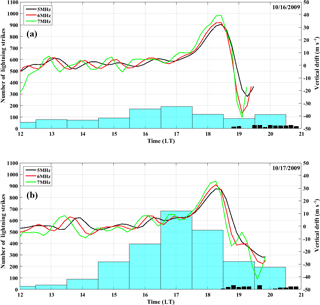
Figure 6Vertical drift (colored lines), lightning strike activity (cyan bars) and the range of spread F, Qf (black bars), for 16 October 2009 (a) and 17 October 2009 (b).
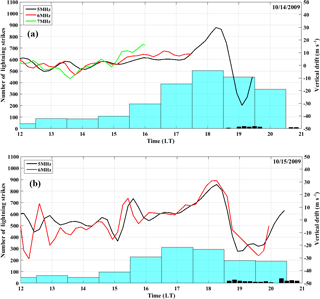
Figure 8Vertical drift (colored lines), lightning strike activity (cyan bars) and the range of spread F, Qf (black bars), for 14 October 2009 (a) and 15 October 2009 (b).
Figure 5 presents the keograms for 15 September 2009 (a) and 18 October 2009 (b), confirming the results presented in the previous figure. In Fig. 5a the complete absence of structures of depletion drifting eastward may be clearly verified for the entire period (18:16–24:56 LT). However, Fig. 5b reveals a completely different result, with EPB structures being registered during a long period (∼19:40–24:00 LT). The severe tropospheric activity on this particular day, as indicated by the lightning strike activity monitoring, causes some interferences in the registers, and clouds eventually covered the area of the sky scanned by the CCD all-sky images, as in the case of the time interval of 21:46–22:51 and after 24:00 LT.
Under similar quiet geomagnetic conditions in equinoctial periods and with equivalent PRVD (and also Vpk) it seems reasonable to argue that the spread-F development on 18 October 2009 was due to a contribution from GWs. Furthermore, the lightning strike activity monitoring is apparently capable of providing an acceptable prognostic of the tropospheric convection and consequently the GW generation and presence in later times.
Both arguments, the external contributions (GWs) and their evaluation through lightning strike activity monitoring are verified in most of the days in our dataset (11–23 September 2009 and 11–23 October 2009). Of course the Vpk threshold requirement is also a delimiter parameter and for several days in September this condition was not attended.
Figure 6 shows a comparison between two consecutive days, 16 October 2009 (a) and 17 October 2009 (b). The PRVD profile for both days is almost similar, actually presenting a lesser value of Vpk in 17 October 2009 (∼35 m s−1) in relation to the value in 16 October 2009 (∼40 m s−1). The lightning strike activity differs considerably, being much more intense on 17 October 2009. An analysis of this figure reveals that the Qf parameter indicates the presence of a spread-F–EPB event earlier on 17 October 2009 (Fig. 6b), even though this day is under the same background conditions and has a lesser Vpk value. Once more, the lightning strike activity monitoring appears to successfully prognosticate this trend of external contribution to seed these spread-F irregularities.
The keograms related to these days (16 and 17 October 2009) are presented in Fig. 7. Figure 7a (16 October 2009) shows some EPB structures starting at ∼19:55 LT and persisting up to 23:49 LT. In Fig. 7b (17 October 2009) the EPB structures appeared considerably earlier (∼18:46 LT) and also remain up to 23:49 LT; additionally, the intensity of the depletion and the number of structures are clearly larger on 17 October 2009, even though the initial conditions (e.g., Vpk) were more favorable on 16 October 2009. Once again, it seems reasonable to argue in terms of external contributions (GW) and one more time the lightning strike activity monitoring had successfully foreseen these features.
One more example is given in Fig. 8 where a comparison between 14 October 2009 (a) and 15 October 2009 (b) is presented. The PRVD profile for both days is similar as well as the Vpk values (∼30 m s−1); the lightning strike activity, however, presented a considerable different intensity. The range of spread F (Qf) registered indicates a similar onset time and duration. It must be said that there was no 7 MHz information on 15 October 2009. The dominant role of the PRVD (and Vpk) and their coincidence for both days would suggest a very similar generation and development of the spread-F–EPB activity, but images registered through the CCD all-sky imager reveal a slightly different scenario.
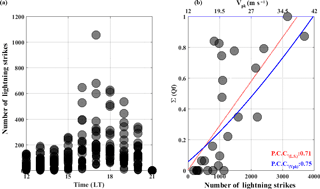
Figure 10Temporal distribution of lightning strikes (a). Distribution of normalized Σ (Qf) as a function of lightning strike activity and the prereversal drift peak Vpk (b).
In Fig. 9 the keograms for the 2 days presented in the previous figure are presented. In Fig. 9a (14 October 2009) the EPB activity started at ∼19:20 LT and multiple structures of severe depletion were manifested up to 00:56 LT. Figure 9b (15 October 2009) exhibits an EPB structure at a considerably later time (∼20:00 LT). This later structure (also broader and slower) is verified until 00:56 LT and secondary structures were registered only after ∼23:28 LT. As was already pointed out, similar results are found for the entire dataset.
MacClure et al. (1998) discussed the coincidence between the location of the intertropical convergence zone (i.e., the region of maximum tropospheric convection) and the occurrence of spread-F events. Röttger (1981, 1980, 1977) presented strong evidence of the relation between thunderstorms, tropospheric convection and the generation and presence of gravity waves that may act as seeders to spread-F–EPB events. Additionally, good correlation was found between average seasonal variations in the ESF occurrence and that of lightning flashes in the region of Cachoeira Paulista (Abdu, 2001).
The results presented in Figs. 4–9 appear to elucidate this vertical coupling between tropospheric convection and ionospheric irregularities. Furthermore the few case studies showing successful prognostics (in the majority of the dataset) on spread-F–EPB occurrence would suggest that the lightning strike monitoring may have the potential to be a viable tool to forecast GW generation and presence and, as a result, the occurrence of EPB structures under favorable scenarios (e.g., when m s−1).
The concentration of the maximum values of lightning strike activity in the months under analysis occurs between 18:00 and 22:00 UT (15:00–19:00 LT); this is shown in Fig. 10a. Gravity waves generated through severe tropospheric convection may present gravitational and acoustic components. The different nature of these contributions implies a propagation time ranging from ∼20 min (acoustic component) up to a few hours (gravitational component) (Kherani et al., 2009, 2011, 2016). The hours in which the maximum lightning strike activity occurs in the dataset represent periods of the day in which gravity waves generated in the troposphere may have an adequate time to reach the ionosphere in the moment of the PRVD action and efficiently seed the instabilities responsible for spread-F events and EPB development.
Figure 10b exhibits the relation between the normalized summation of the Qf parameter for each of the 26 days in the dataset in the time interval from 21:00 to 24:00 UT (18:00–21:00 LT) and the summation of the lightning strike activity for the same days, in the time interval of 15:00–24:00 UT (12:00–21:00 LT). The linear relation between these quantities reveals a reasonable Pearson correlation coefficient (0.71), indicating an implicit association as verified in the analyses throughout this work. Additionally, on the blue line, the values of the peak of vertical drift during the prereversal enhancement (Vpk) are presented. The Pearson correlation coefficient between these values as well as the normalized summation of Qf values is about 0.75. The relation between this parameter (i.e., Vpk) and the development of spread-F–EPB structures is well known (Fejer et al., 1999) and since this value of correlation is similar to that obtained with lightning strike activity, this seems to complement the analysis proposed in this work.
In this work a comparative investigation of the lightning strike activity and the presence and generation of spread-F–EPB structures was presented. The selected days present similar seasonal (September–October equinox) and geomagnetic conditions (Kp ≤4), and the dataset is a continuous temporal series during a deep solar minimum (2009). This was done in an attempt to present similar background conditions. However, some factors may be the slightly different, such as for instance the strength of the bottom-side plasma density gradient, plasma density distribution, neutral winds, etc. Nevertheless, it was assumed that these contributions could be negligible due to the specific criterion adopted in the data selection. It is generally accepted that the lightning strike activity is closely related to severe tropospheric convection (Deierling and Petersen, 2008; Boccippio, 2001; Williams et al., 1989 and references therein). Moreover, strong tropospheric convection is usually evoked to explain the generation of gravity waves (with acoustic and gravitational components) (e.g., Li et al., 2016; Kherani et al., 2009, 2011, 2016; Dutta et al., 2009; Fritts et al., 2008; Hoke and Tsuda, 2001; Lane et al., 2001; Dewan et al., 1998; Taylor and Hapgood, 1988; Röttger, 1977, 1980, 1981). The results presented throughout this work suggest that the lightning strike activity monitoring may provide valuable prognostic information about GW generation and presence, since the common source of both (namely, severe tropospheric convection) seems to be properly evaluated, indirectly, through information on the number of lightning strikes. Previous knowledge about day-to-day GW activity is highly desirable since these waves are potential candidates to seed irregularities on the ionospheric plasma. The results presented here indicate that for a forecast approach, the knowledge of day-to-day lightning strike activity may provide a considerable contribution, once it is possible to obtain this information from dozens of minutes up to a few hours before the GWs reach the ionosphere and trigger instabilities.
The key points of the present investigation may be listed as follows:
-
The PRVD (Vpk) plays a fundamental role in the generation and development of spread-F–EPB events. For considerably small values of Vpk the external contribution of GWs is not enough to trigger instabilities.
-
For equivalent Vpk threshold conditions (e.g., Vpk∼20 m s−1), days with a larger number of lightning strike activity are coincident with the presence of spread-F–EPB structures. However, days with a considerably smaller number of lightning strikes are coincident with the complete absence of spread-F–EPB activity. The similar Vpk conditions seem to support the argument that some external contribution (presumably GW) was crucial for the different characteristics verified in the spread-F–EPB activity, and the lightning strikes appear to be a key factor that contributed to the observed difference in the characteristics.
-
For similar geomagnetic and seasonal conditions and with equivalent PRVD (Vpk) magnitudes, it was found that in days with considerably larger activity of lightning strikes, spread-F–EPB structure generation occurred substantially earlier and with some distinct characteristics. Once again, the similarity of the Vpk magnitude and geomagnetic activity would suggest that an external contribution, probably from gravity waves, could be responsible for the different characteristics in spread F–EPB observed afterwards. One more time, the lightning strike activity seems to be indicative of the convection intensity and hence the strength of the GW activity that in turn presented agreement with the spread F–EPB occurring at later times.
-
The lightning strike dataset reveals a concentration of maximum activity between 15:00 and 19:00 LT, an adequate time for the generation of GW capable of reaching the ionosphere in the nearness of the PRVD time, when the rise of the ionosphere provides an unstable scenario in which instabilities may grow. Furthermore, an analysis of the implicit relation of Qf (range of the spread-F trace) and the number of lightning strikes exhibits a considerable correlation coefficient (0.71), similar to the correlation found with the Vpk, indicating thereby that the usage of lightning strike activity may provide a qualitative and complementary parameter together with Vpk in forecast approaches.
The Digisonde and CCD all-sky data used in this work may be made available by contacting Inez Staciarini Batista (inez.batista@inpe.br) and José Humberto Andrade Sobral (jhasobral@yahoo.com.br), respectively.
JS, JHAS, EAK, MMFS and DRdC analyzed the data, JS, JHAS and EAK prepared the paper.
The authors declare that they have no conflict of interest.
This article is part of the special issue “Space weather connections to near-Earth space and the atmosphere”. It is a result of the 6∘ Simpósio Brasileiro de Geofísica Espacial e Aeronomia (SBGEA), Jataí, Brazil, 26–30 September 2016.
The authors wish to thank the World Wide Lightning Location Network (http://wwlln.net), a collaboration among over 50 universities and institutions,
for providing the lightning location data used in this paper, as well as Robert Holzworth and Craig J. Rodger. José Humberto Andrade
Sobral and Esfhan Alam Kherani are grateful to the support from Conselho Nacional de Desenvolvimento Científico e Tecnológico (CNPq)
through grants 303741/2014-7 and 307496/2015-5, respectively, and Marcelo Magalhães Fares Saba acknowledges FAPESP support through grant 2012/15375-7.
Authors also acknowledge Inez Staciarini Batista for the Digisonde data.
The topical editor, Mangalathayil Abdu, thanks two
anonymous referees for help in evaluating this paper.
Abdu, M. A.: Outstanding problems in the equatorial ionosphere-thermosphere system relevant to spread F, J. Atmos. Sol.-Terr. Phy., 63, 869–884, 2001.
Abdu, M. A.: Equatorial spread F/plasma bubble irregularities under storm time disturbance electric fields, J. Atmos. Sol.-Terr. Phy., 75–76, 44–56, 2012.
Abdu, M. A., Batista, I. S., and Sobral, J. H. A.: Equatorial ionospheric plasma bubble irregularity occurrence and zonal velocities under quiet and disturbed conditions from polarimeter observations, J. Geophys. Res., 90, 9921–9928, 1985.
Abdu, M. A., Sastri, J. H., Dougall, J. M., Batista, I. S., and Sobral, J. H. A.: Equatorial disturbance dynamo electric field, longitude structure and spread-F: a case study from Guara/EITS Campaigns, Geophys. Res. Lett., 24, 1707–1710, 1997.
Abdu, M. A., Alam Kherani, E., Batista, I. S., de Paula, E. R., Fritts, D. C., and Sobral, J. H. A.: Gravity wave initiation of equatorial spread F/plasma bubble irregularities based on observational data from the SpreadFEx campaign, Ann. Geophys., 27, 2607–2622, https://doi.org/10.5194/angeo-27-2607-2009, 2009a.
Abdu, M. A., Kherani, E. A., Batista, I. S., and Sobral, J. H. A.: Equatorial evening prereversal vertical drift and spread F suppression by disturbance penetration electric fields, Geophys. Res. Lett., 36, L19103, https://doi.org/10.1029/2009GL039919, 2009b.
Abdu, M. A., Batista, I. S., Reinisch, B. W., de Souza, J. R., Sobral, J. H. A., Pedersen, T. R., Medeiros, A. F., Schuch, N. J., de Paula, E. R., and Groves, K. M.: Conjugate Point Equatorial Experiment (COPEX) campaign in Brazil: electrodynamics highlights on spread F development conditions and day-to-day variability, J. Geophys. Res., 114, A04308, https://doi.org/10.1029/2008JA013749, 2009c.
Abdu, M. A., Batista, I. S., Reinisch, B. W., MacDougall, J. W., Kherani, E. A., and Sobral, J. H. A.: Equatorial range spread F echoes from coherent backscatter, and irregularity growth processes, from conjugate point digital ionograms, Radio Sci., 47, 1–8, https://doi.org/10.1029/2012RS005002, 2012.
Alam Kherani, E., Abdu, M. A., de Paula, E. R., Fritts, D. C., Sobral, J. H. A., and de Meneses Jr., F. C.: The impact of gravity waves rising from convection in the lower atmosphere on the generation and nonlinear evolution of equatorial bubble, Ann. Geophys., 27, 1657–1668, https://doi.org/10.5194/angeo-27-1657-2009, 2009a.
Boccippio, D. J.: Lightning scaling relations revisited, J. Atmos. Sci., 59, 1086–1104, 2001.
Deierling, W. and Petersen, W. A.: Total lightning activity as an indicator of updraft characteristics, J. Geophys. Res.-Atmos., 113, D16210, https://doi.org/10.1029/2007JD009598, 2008.
Dewan, E. M., Picard, R. H., O'Neil, R. R., Gardiner, H. A., Gibson, J., Mill, J. D., Richards, E., Kendra, M., and Gallery, W. O.: MSX satellite observations of thunderstorm-generated gravity waves in mid-wave infrared images of the upper stratosphere, Geophys. Res. Lett., 25, 939–942, 1998.
Dutta, G., Kumar, M. C. A., Kumar, P. V., Ratnam, M. V., Chandrashekar, M., Shibagaki, Y., Salauddin, M., and Basha, H. A.: Characteristics of high-frequency gravity waves generated by tropical deep convection: case studies, J. Geophys. Res., 114, D18109, https://doi.org/10.1029/2008JD011332, 2009.
Fejer, B. G., Scherliess, L., and de Paula, E. R.: Effects of the vertical plasma drift velocity on the generation and evolution of equatorial spread F, J. Geophys. Res., 104, 19859–19869, 1999.
Fritts, D. C. and Vadas, S. L.: Gravity wave penetration into the thermosphere: sensitivity to solar cycle variations and mean winds, Ann. Geophys., 26, 3841–3861, https://doi.org/10.5194/angeo-26-3841-2008, 2008.
Fritts, D. C., Vadas, S. L., Riggin, D. M., Abdu, M. A., Batista, I. S., Takahashi, H., Medeiros, A., Kamalabadi, F., Liu, H.-L., Fejer, B. G., and Taylor, M. J.: Gravity wave and tidal influences on equatorial spread F based on observations during the Spread F Experiment (SpreadFEx), Ann. Geophys., 26, 3235–3252, https://doi.org/10.5194/angeo-26-3235-2008, 2008.
Hoke, K. and Tsuda, T.: Gravity waves and ionospheric irregularities over tropical convection zones observed by GPS/MET radio occultation, Geophys. Res. Lett., 28, 2815–2818, 2001.
Huang, C. S. and Kelley, M. C.: Nonlinear evolution of equatorial spread F: 1. On the role of plasma instabilities and spatial resonance associated with gravity wave seeding, J. Geophys. Res., 101, 283–292, 1996a.
Huang, C. S. and Kelley, M. C.: Nonlinear evolution of equatorial spread F: 2. Gravity wave seeding of Rayleigh–Taylor instability, J. Geophys. Res., 101, 293–302, 1996b.
Huang, C. S. and Kelley, M. C.: Nonlinear evolution of equatorial spread F: 3. Plasma bubbles generated by structured electric fields, J. Geophys. Res., 101, 303–313, 1996c.
Huang, C. S. and Kelley, M. C.: Nonlinear evolution of equatorial spread F: 4. Gravity waves, velocity shear, and day-to-day variability, J. Geophys. Res., 101, 24521–24532, 1996d.
Huang, C. S., Kelley, M. C., and Hysell, D. L.: Nonlinear Rayleigh–Taylor instabilities, atmospheric gravity waves and equatorial spread F, J. Geophys. Res., 98, 15631–15642, 1993.
Hysell, D. L., Kelley, M. C., Swartz, W. E., and Woodman, R. F.: Seeding and layering of equatorial spread F by gravity waves, J. Geophys. Res., 95, 17253–17260, https://doi.org/10.1029/JA095iA10p17253, 1990.
Kelley, M. C., Larsen, M. F., LaHoz, C., and McClure, J. P.: Gravity wave initiation of equatorial spread F: a case study, J. Geophys. Res., 86, 9087–9100, 1981.
Kherani, E. A. and Abdu, M. A.: The acoustic gravity wave induced disturbances in the equatorial ionosphere, in: Aeronomy of the Earth's Atmosphere and Ionosphere, edited by: IAGA/IUGG Springer, Vol. 1, 1st Edn., Springer, Dordrecht, 141–162, 2011.
Kherani, E. A., Rolland, L., Lognonné, P., Sladen, A., Klausner, V., and de Paula, E. R.: Traveling ionospheric disturbances propagating ahead of the Tohoku-Oki tsunami: a case study, Geophys. J. Int., 204, 1148–1158, https://doi.org/10.1093/gji/ggv500, 2016.
Lane, T. P., Reeder, M. J., and Clark, T. L.: Numerical modeling of gravity wave generation by deep tropical convection, J. Atmos. Sci., 58, 1240–1274, 2001.
Li, G., Otsuka, Y., Ning, B., Abdu, M. A., Yamamoto, M., Wan, W., Liu, L., and Abadi, P.: Enhanced ionospheric plasma bubble generation in more active ITC Z, Geophys. Res. Lett., 121, 2389–2395, 2016.
Makela, J. J., Vadas, S. L., Muryanto, R., Dulty, T., and Crowley, G.: Periodic spacing between consecutive equatorial plasma bubbles, Geophys. Res. Lett., 37, L14103, https://doi.org/10.1029/2010GL043968, 2010.
McClure, J. P., Singh, S., Bamgboye, D. K., Johnson, F. S., and Kil, H.: Occurrence of equatorial F region irregularities: evidence for tropospheric seeding, J. Geophys. Res., 103, 29119–29135, 1998.
Röttger, J.: Travelling disturbances in the equatorial ionosphere and their association with penetrative cumulus convection, J. Atmos. Sol.-Terr. Phy., 39, 987–998, 1977.
Röttger, J.: Structure and dynamics of the stratophere and mesosphere revealed by VHF radar investigations, Pure Appl. Geophys., 118, 494–527, 1980.
Röttger, J.: Equatorial spread-F by electric fields and atmospheric gravity waves generated by thunderstorms, J. Atmos. Sol.-Terr. Phy., 43, 453–462, 1981.
Röttger, J.: Gravity waves seeding ionospheric irregularities, Nature, 296, 111–112, 1982.
Singh, S., Johnson, F. S., and Power, R. A.: Gravity wave seeding of equatorial plasma bubbles, J. Geophys. Res.-Space, 102, 7399–7410, 1997.
Sobral, J. H. A. and Abdu, M. A.: Latitudinal gradient in the plasma bubble zonal velocities as observed by scanning 630 mm airglow measurements, J. Geophys. Res., 95, 8253–8257, 1990.
Sobral, J. H. A. and Abdu, M. A.: Solar activity effects on equatorial plasma bubble zonal velocity and its latitude gradient as measured by airglow scanning photometers, J. Atmos. Sol.-Terr. Phy., 53, 729–742, 1991.
Sobral, J. H. A., Abdu, M. A., Gonzalez, W. D., Tsurutani, B. T., and Batista, I. S.: Effects of intense storms and subtorms on the equatorial ionosphere/thermosphere system in the American sector from ground based and satellite data, J. Geophys. Res., 102, 14305–14313, 1997.
Sobral, J. H. A., Abdu, M. A., Yamashita, C. S., Gonzalez, W. D., Gonzalez, A. L. C., Batista, I. S., Zamlutti, C. J., and Tsurutani, B. T.: Responses of the low-latitude ionosphere to very intense geomagnetic storms, J. Atmos. Sol.-Terr. Phy., 63, 965–974, 2001.
Sobral, J. H. A., Abdu, M. A., Takahashi, H., Taylor, M. J., de Paula, E. R., Zamlutti, C. J., Aquino, M. G., and Borba, G. L.: Ionospheric plasma bubble climatology over Brazil for a Period of Observation of 22 Years (1977–1998), J. Atmos. Sol.-Terr. Phy., 64, 1517–1524, 2002.
Sousasantos, J., Kherani, E. A., and Sobral, J. H. A.: A numerical simulation study of the collisional-interchange instability seeded by the pre-reversal vertical drift, J. Geophys. Res., 118, 7438–7449, 2013.
Sousasantos, J., Kherani, E. A., and Sobral, J. H. A.: An alternative possibility to equatorial plasma bubble forecasting through mathematical modeling and Digisonde data, J. Geophys. Res., 102, 1–12, 2017.
Taylor, M. J. and Hapgood, M. A.: Identification of a thunderstorm as a source of short period gravity waves in the upper atmospheric nightglow emissions, Planet. Space Sci., 36, 975–985, 1988.
Vadas, S. L. and Fritts, D. C.: Reconstruction of the gravity wave field from convective plumes via ray tracing, Ann. Geophys., 27, 147–177, https://doi.org/10.5194/angeo-27-147-2009, 2009.
Williams, E. R., Weber, M. E., and Orville, R. E.: The relationship between lightning type and convective state of thunderclouds, J. Geophys. Res.-Atmos., 94, 13213–13220, https://doi.org/10.1029/JD094iD11p13213, 1989.